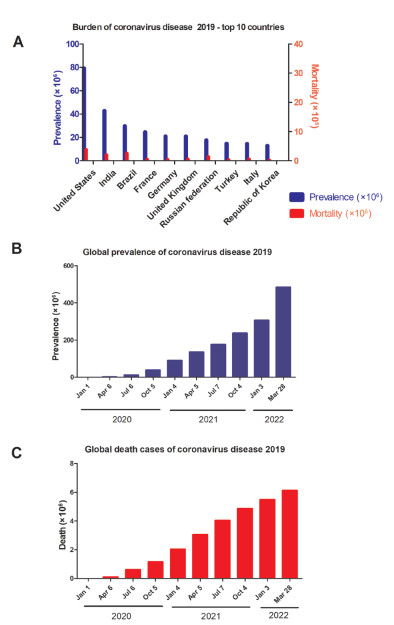
Citation: | Weijie Guan, Jianxing He. Clinical characteristics, management, and prevention of coronavirus disease 2019[J]. Frigid Zone Medicine, 2023, 3(3): 134-160. doi: 10.2478/fzm-2023-0019 |
Coronaviruses belong to a group of the single-stranded RNA viruses that have been associated with respiratory tract infections. Three major outbreaks of severe acute respiratory diseases associated with coronavirus infections occurred during the 20th century. The first outbreak was caused by the severe acute respiratory syndrome (SARS) coronavirus, which resulted in 8, 422 laboratory-confirmed cases and 919 deaths worldwide[1]. The second outbreak, caused by the Middle East Respiratory Syndrome (MERS) coronavirus, was less prevalent (2 254 cases globally as of 2018) but had a higher fatality rate (35.5%)[2]. However, these outbreaks were dwarfed in scale and socioeconomic impact by of the ongoing COVID-19 pandemic caused by severe acute respiratory syndrome coronavirus 2 (SARS-CoV-2). However, these outbreaks were dwarfed in scale and socioeconomic impact by the ongoing COVID-19 pandemic caused by severe acute respiratory syndrome coronavirus 2 (SARS-CoV-2).
Since December 2019, the number of laboratory-confirmed cases has rapidly increased with over 600 million cases and 6 million death reported as of December 8th, 2022, and the trend of increase persists[3]. The prevalence of cases varied substantially across different countries and continents with global efforts focused on contact tracing, early diagnosis, and isolation, and controlling infections at borders to contain the spread of the virus. However, the implementation of lockdowns in several countries has resulted in secondary or tertiary outbreaks due to the loosening of preventative measures. The COVID-19 pandemic has caused unprecedented global economic burden, including city and country lockdowns, closures of work and schools, and the cancellation of public transportation and social activities. These changes have led to an upsurge of medical expenditures, insufficient supplies of daily necessities, unemployment, and increased inequity, which may lead to social chaos and conflicts.
Therefore, there is a pressing need to develop new drugs and vaccines to combat COVID-19, which relies on a better understanding of the molecular structures of SARS-CoV-2 and the pathobiology of the disease. However, the timeline and cost of developing new drugs de novo is substantial, and so the assessment of efficacy and safety of repurposed drugs has been prioritized, but few have been proven effective against SARS-CoV-2 infections. Mass vaccination has become a top priority to minimize the incidence of infections until herd vaccination is achieved, and maintaining social distancing, contact tracing, and strengthening personal protection remain indispensable. In this review, we highlight the epidemiology, pathophysiology, prognosis, diagnostics, and therapeutics of COVID-19, while addressing the gaps in scientific research and the challenges in scaling up global preparedness.
The rapid spread and global scale of transmission are two defining characteristics of the epidemiology of COVID-19. Since the early stage of the outbreak, enormous efforts have been made globally to understand the mode of transmission and curb its spread at national and sub-national levels.
The first documented symptomatic case of COVID-19 was identified in Wuhan, China, in December 2019[4]. Subsequently, cases with similar clinical manifestations were soon identified, and the emergence of several clusters of cases, including some among family members, provided the solid evidence for human-to-human transmission. Sequencing studies confirmed that SARS-CoV-2 shared a substantial similarity in nucleic acid sequences compared with SARS-CoV and the bat coronavirus. However, these similarity analyses could not convincingly indicate the origins of the virus. It remains unclear whether bats or pangolins were the dominant species directly responsible for human infections. Earlier sporadic transmissions in other countries or continents cannot be excluded. Further global efforts are required to fully ascertain the origins of the virus[5].
In December 2019, most cases had a direct contact with the Huanan Seafood market, whereas less than 10% reported the same since January 2020[6]. These cases, along with the clusters of cases[7], provided the early evidence of human-to-human transmission. The median latency period was approximately 7 days[8], and the median basic reproductive number was 2.2 in the early stages, which was higher than that of SARS and MERS[6]. The number of laboratory-confirmed cases surged in different countries and regions, mainly in North and South America and Europe. The US, Brazil, and India have reported the largest number of laboratory-confirmed cases[1] (Fig. 1). Despite the implementation of preventative measures and vaccination programs, several waves of outbreaks occurred possibly due to the loosening of these measures and the emergence of new variants.
There have been concerns regarding the impact of low temperature on the infectivity of SARS-CoV-2. A study on hamsters have revealed that low temperature can enhance virus shedding, increase disease severity, trigger proinflammatory cytokine/chemokine expression, and decrease neutralizing antibody responses[9]. Research has also shown that temperature has a positive association with the incidence of COVID-19, and the vice versa[10]. Moreover, several studies have suggested that the relationship between temperature and mortality risk from COVID-19 is non-linear, and a U-shaped association may exist[11-13].
Reduction of direct interpersonal contact and minimizing case importation or exportation remain at the core of population-based preventive strategies for communicable diseases. In the early stage of outbreak in Wuhan, travel restrictions of only 3-5 days reduced the incidence of cases by 80%[14]. While consumer-grade masks provide equivalent or better protective effects compared with the non-N95 medical masks[15], a combination of wearing masks, canceling school or work, mass testing, strict quarantine, and social distancing more efficiently contained in Singapore and China than implementing single measures alone[16-17]. Both China and Singapore adopted aggressive containment measures, consisting mainly of track and tracing, early isolation of cases, proactive community-based screening and early interventions (i.e., early admission to Fangcang shelter hospitals)[18]. In late January 2020, the World Health Organization (WHO) declared the outbreak of Covid-19 as a Public Health Event of International Concern (PHEIC), an event that is deemed to be extraordinary, constitute a public health risk to other states through international spread of disease, and potentially require a coordinated international response. However, the announcement of PHEIC should be based on a careful evaluation of both the geographic extension and severity of illness. Considerations should center on how to balance the economic growth with the life and livelihood of people. An over-reacted declaration of PHEIC during the H1N1 influenza pandemic has been associated with significant economic loss (e.g., intensive travel restriction, closure of business trades), and social panic. Nevertheless, the current grading of PHEIC would not substantially benefit from adding an intermediate level, which would elicit confusion regarding whether to implement aggressive measures at national or sub-national levels.
Despite the extensive use of polymerase chain reaction (PCR) assays, there remains a substantial number of undocumented infections which contribute to the rapid spread of COVID-19[19]. Seropositivity rate vary considerably among different countries or regions: 17.1% in 18 cities of Iran[20], 10.8% in Geneva, Switzerland[21], 10.6% among asymptomatic and 44.7% among symptomatic healthcare workers in the UK[22], 3.2%-3.8% in Wuhan, China[23], 6.9% in mainland China[24], 1%-23% at jurisdiction levels in the US[25], and approximately 2.8% in Brazil[26]. These findings indicated that developing herd immunity through natural infections would hardly suffice and that proactive implementation of vaccination programs is necessary.
Given the rapidly evolving epidemiology and enormous socioeconomic burden, it is essential to have a better understanding of the pathophysiology of SARS-CoV-2 infections to explore key targets for interventions. Figure 2 illustrates the primary pathophysiological mechanism of SARS-CoV-2 infections within the airways.
SARS-CoV-2 shared a nucleotide sequence identity of 79.6% to 90% with SARS-CoV[4, 27-29]. The spike protein plays a crucial role in the susceptibility to SARS-CoV-2 infections, as it contains the receptor binding domain[29-30]. Both the receptor binding domain and N-terminal domain are key molecular targets for interventions with neutralizing antibodies[31-32]. Co-factors of ACE2, including transmembrane serine protease 2 and furin[33], are enriched in alveolar type 2 cells and macrophages[34], and therefore may also be potential targets for therapeutics.
Human ACE2 transgenic mice models have been constructed to recapitulate the pathological changes observed in human, including severe pneumonia leading to fatal respiratory diseases[35-38]. Reverse genetics has been successfully applied to generate the recombinant mouse-adapted SARS-CoV-2, which utilizes the mouse ACE2 for cellular entry[39]. The main advantage of the ACE2 transgenic mice model is that it produces markedly higher viral titers in the inferior turbinate and lungs and robust cytokines/chemokines production compared to the conventional adenovirus mice model[40]. Additionally, a non-transgenic mice model, which uses the replication-deficient adenovirus 5 with the ACE2 sequence, elicits severe pulmonary pathology and high viral titers in the lungs[41]. Rhesus macaques are more susceptible to SARS-CoV-2 infection compared to the mouse models, and the typical pathologic manifestations they exhibit (i.e., pulmonary infiltrate, marked viral shedding) recapitulates the respiratory diseases observed in humans. Thus, they provide an ideal model for mechanistic investigations of SARS-CoV-2 infections[42]. Moreover, rhesus macaques were valuable to test the natural immunity against re-infections[43-44], and the efficacy of adenovirus vaccines[45], and DNA vaccines[46]. Finally, the hamster model of SARS-CoV-2 infection helps reveal the pharmacologic actions of clofazimine combined with remdesivir[47].
The typical pathologic manifestations of COVID-19 include mucus hypersecretion, formation of neutrophil extracellular traps, and diffuse alveolar damage, which includes hyaline membrane formation, fibrinous exudation, and organizing fibrosis[48-49]. Other important pulmonary lesions include thrombosis and microangiopathy in small vessels and capillaries[48, 50-51]. COVID-19 should also be regarded as a systemic disease, as it can affect organs such as heart, kidney, brain, and gastrointestinal tract. Cardiac lesions can present with CD4+, CD8+, and CD31+ lymphocyte infiltration, while renal lesions can present with tubular dilation and loss of tubular epithelial cell brush borders. Cerebral lesions can present with eosinophilia, nuclear and cytoplasmic condensation of neurons, and lymphocyte infiltration[48, 52-53].
There is a delicate immunoregulatory mechanism that fine-tunes the immune responses in patients with mild-to-moderate COVID-19. However, the disrupted airway epithelial-endothelial barrier and the secondary injury by infiltrating neutrophils and monocytes, patients with severe COVID-19 may cause an exaggerated macrophage-mediated inflammatory response in patients with severe COVID-19[54]. Defective type I interferon responses have been linked to severe COVID-19[55-56]. The severe end of viral sepsis may result from cytokine-induced collateral damage due to prolonged activation of signaling pathways such as nuclear factor κB, mitogen-activated protein kinase, Janus kinase-signal transducer, activator of transcription 3, and mammalian target of rapamycin. Collectively, this may lead to the exuberant systemic inflammatory responses and secondary multiorgan failure[57-58].
Pharyngeal swab has been the preferred sampling technique for PCR assays of SARS-CoV-2 because of the high viral loads that peak within the first week of infection and waned thereafter[59-60]. However, viral loads do not differ between mild and severe disease[61]. Both throat washing and salivary sampling are patient-friendly alternatives that yield similar or higher detection rates of SARS-CoV-2 compared to pharyngeal swabs[62-63]. Anterior nasal swabbing for rapid antigen testing has also become increasingly adopted worldwide.
Viral shedding lasts longer with a median duration of ~17.0 days in the upper respiratory tract), ~16.6 days in serum, and ~17.2 days in gastrointestinal tract than in the lower respiratory tract (~14.6 days)[64]. However, shedding of live viruses can be short-lived[64] and restricted to the respiratory tract in patients with mild diseases[59, 65]. A minority of patients with common-type or severe COVID-19 may have prolonged SARS-CoV-2 positivity, possibly due to viral fragment excretion that does not have transmissibility[66].
The viral load kinetics correlate with the antibody responses that are indicative of the protective effects against re-infections[63]. There is a cross-reactivity of antibodies to SARS-CoV and SARS-CoV-2[65]. The dynamics of IgG and IgM vary considerably over time among individual patients. IgM levels peak before day 20, and IgG levels peak after day 30[67-68]. Although IgG responses are more constant over time (except for the lower levels in asymptomatic patients)[68], IgM responses are more prominent in severe diseases[65]. Seroconversion of IgM and IgG occurs either sequentially or simultaneously[68] (Fig. 3). IgA levels are also elevated during the early stage, especially in severe cases but decreased after one month of symptom onset though remained detectable in saliva for a long time[69-70]. Thus, these assays complement in aiding the diagnosis of COVID-19[71].
Cellular immunity is crucial to mount an adequate immune response to SARS-CoV-2. Among severe cases, T-cell receptor repertoires are significantly diminished at symptom onset followed but recover at convalescence, and the clonal expansion of B cells with decreased diversity occurs at convalescence[72]. There is a defective interferon response, down-regulation of human leukocyte antigen Ⅱ expression, and abnormal neutrophilic inflammation among patients with severe COVID-19[73]. Patients with severe COVID-19 have up-regulated expression of chemokines in monocyte-derived macrophages and down-regulated expression of human leukocyte antigen class Ⅱ[74]. Within the alveoli, an expansion of pro-inflammatory monocyte-derived macrophage is identified in patients with severe COVID-19[74], whereas clonal expansion of CD8+ T cells is seen in patients with moderate COVID-19[75].
The error-prone nature of nucleic acid replication has led to the emergence of SARS-CoV-2 variants early in the outbreak. Some mutations have resulted in amino acid alterations in the receptor-binding domain and N-terminal domain[76], leading to the S gene target failure[77]. Concerns have been raised about the lack of natural immunity against the variants among the whole population. The B.1.1.7 variant emerged in the UK and has been associated with greater transmissibility and higher viral loads[77-78], but did not result in greater disease severity[79]. The B.1.429 variant, which has three spike protein mutations, was rapidly circulating in California in 2021[80]. The B.1.351 variant, characterized by its ability to escape immunity[81-82], and the P.1 variant, which had higher transmissibility and immune escape ability[83], have also emerged. More recently, the Omicron variant has caused further global transmission waves due to its markedly increased infectivity, although disease severity appeared to be lower than the Delta variant after taking into account the confounding effect of vaccination and waning immunity[84].
The impact of emerging variants on pathophysiology and vaccination outcomes has been substantial. Compared to the canonical strains isolated from Wuhan, the B.1.1.7, B.1.351 and B.1.429 variants have been associated with a reduced vaccine efficacy (e.g., mRNA-1273, NVX-CoV2373 and ChAdOx1 nCoV-19 vaccines)[85-88]. Although protective effects of the two-dose regimen of ChAdOx1 nCoV-19 vaccine against mild-to-moderate COVID-19 due to the B.1.351 variant were not observed[89], vaccine efficacy against the B.1.1.7 variants mostly exceeded 50% in recent clinical trials[75, 85-86]. Furthermore, neutralizing antibody responses against both the B.1.351 strain and P.1 strain were cross-reactive[90], and a single dose of the BNT162b2 vaccine (i.e., BNT162b2) elicited a robust antibody response against the B.1.1.7, B.1.351 and P.1 variants[91-92]. However, a marked decrease (up to 35-fold reduction) in the protective effect of vaccines (e.g., mRNA1273, ChAdOx1 nCoV-19, and BNT162b2) against the Omicron variant has been observed[93-94], although a fourth dose of vaccine would not provide further incremental protection[95]. All of this suggests a blunted yet acceptable protective effect against the variants of concern other than the Omicron variant.
SARS-CoV-2 infection triggers immune responses that protect against future infections. However, natural immunity wanes over time and that varies among individuals[96], raising concerns about the durability of immunity and the risk of re-infections. A Danish population-based study found that the rate of protection against re-infection was 80.5% in the general population, but only 47.1% in patients aged 65 years or older[97]. A British study documented that healthcare workers previously infected with SARS-CoV-2 had a 84% reduced risk of acquiring re-infections, and this protective effect persisted for at least 7 months[98]. Among seropositive young adults, the viral loads were 10-fold lower, and the risk of re-infection was 80% lower compared with seronegative individuals[99]. Nonetheless, re-infection can still occur in a minority of patients, and further research is needed to understand the underlying mechanisms.
The heterogeneity of clinical manifestations of COVID-19 has underscored the importance of exploring clinical variables with prognostic implications. By understanding the various clinical presentations, we can uncover key factors that may inform therapeutic interventions.
Respiratory symptoms, including cough, dyspnea, and fever, as well as systemic symptoms such as fatigue, malaise, hyposmia, dysgeusia, have been reported in some patients[8, 100-101]. Nearly 50% of patients remained afebrile on admission[8]. In children and adolescents, COVID-19 can cause life-threatening multisystem inflammatory syndrome, manifested mainly as gastrointestinal, cardiovascular, hematologic and mucocutaneous disorders[102]. Radiologic abnormalities may be absent upon screening in some PCR-positive symptomatic patients[8]. Ground-glass opacity and patchy shadowing are seen in over 50% of patients upon admission[8], which can rapidly progress to diffuse ground-glass opacity, consolidation, or mixed patterns[103]. Apart from lymphopenia, which is significantly correlated with the prognosis[8], other laboratory abnormalities include neutropenia, thrombocytopenia, elevated levels of C-reactive protein, alanine aminotransferase, aspartate aminotransferase, creatine kinase, and d-dimer[8, 101, 104]. In patients with cytokine release syndrome, elevated levels of ferritin can help discriminate survivors from non-survivors[104].
Asymptomatic patients can be divided into two categories - those who remain completely asymptomatic throughout the course of disease and those whose symptoms are not detected during screening or testing. Pooled data of the general population indicates that approximately 20% to 75% of patients remained asymptomatic upon testing[105]. Asymptomatic patients have a weaker immune response, as evidenced by lower IgG titer and neutralizing antibody levels[106]. However, these patients had similar viral loads compared to symptomatic individuals, raising concerns about the equivalent probability of environmental contamination[107]. It is important to note that asymptomatic patients may constitute the majority of patient populations in communities[108], which can explain the rapid global spread of the virus.
COVID-19 has affected people of all ages, younger patients without comorbidity are less likely to develop severe or critical illness[109]. However, in an ageing society, the association between comorbidities and the adverse outcomes of COVID-19 cannot be underestimated. Older patients have a higher prevalence of comorbidities[110], blunted immune responses to SARS-CoV-2[111-112], and poor clinical outcomes[113-114]. Around 25% of patients had at least one comorbidity[115], such as hypertension, diabetes, cardiovascular diseases, chronic obstructive pulmonary disease (COPD), and chronic kidney diseases[104, 115]. Although most of these comorbidities predispose patients to poor clinical outcomes[104, 115-116], there are still controversies regarding the impact of chronic respiratory diseases, such as asthma, on COVID-19[117-121].
There are variations in the definitions of COVID-19 severity. For instance, the 5th WHO interim report categorized COVID-19 as mild, moderate and critical based on symptoms, radiologic characteristics, and resting oxygen saturation (with a cut-off at 90%)[122]. In contrast, the Chinese clinical guidelines defined severe disease based on respiratory rate (with a cut-off at 30 per minute), dyspnea, and resting oxygen saturation (with a cut-off at 94%)[123]. Moreover, there is no consensus on the optimal scoring system for severity rating of COVID-19. The rating for grading severity, which has been adopted in many clinical trials, was extrapolated from the WHO-recommended grading system for influenza (typically 7- or 8-point grading)[124].
Several variables, including age, lymphopenia, interleukin-6, interleukin-8, and ferritin, can provide prognostic indications for COVID-19 outcomes[104, 125-126]. Various proteins (such as serum amyloid protein) and metabolites (such as choline, bilirubin, and sphingolipids)[127], as well as the degree of airway dysbiosis[128], can identify patients with severe COVID-19. In clinical practice, the development of a simple model integrating age, respiratory and systemic symptoms, comorbidities, and laboratory test findings has achieved accurate predictions of disease progression[129]. This integrated model performs better than other conventional metrics, such as CURB-65. Further validation of a simple, integrated score for prognostication is needed.
Timely and accurate diagnosis is crucial to identify patients who might cause community dissemination. While PCR assays are currently the gold standard for conclusive diagnosis, developing rapid and more accurate tests could further improve diagnosis and promote these methods to the forefront of clinical application.
The surge in COVID-19 cases and the need for prompt triage of patients have led to the development of rapid laboratory diagnostics. Rapid antigen testing can detect cases, close contacts, and asymptomatic individuals which high viral loads[130]. Loop-mediated isothermal amplification technology yielded 100% sensitivity and 97.6% specificity in detecting SARS-CoV-2[131]. A high-throughput multi-index isothermal amplification platform significantly reduced the processing time (16 samples detected in a single run within 90 min) and achieved a high concordance (98.15%) with PCR assays[132]. This can be accelerated by a highly automated mobile laboratory for on-site rapid diagnosis[133]. Rapid diagnostic tests based on clustered regularly interspaced short palindromic repeats can also detect the virus within 15 minutes with minimal hands-on time[134].
Point-of-care testing has largely reduced waiting time (median: 1.7 hrs vs. 21.3 hrs for PCR-based assays) and decentralize testing in busy laboratories[135], and rapid antigen-based assays can be readily incorporated into the current testing algorithm in developing countries[67]. Recently, advances have enabled lab-free point-of-care testing of viral nucleic acids with a single cartridge that integrated seven SARS-CoV-2 gene targets, providing a sensitive and specific means to enable rapid clinical decisions[136].
Pneumonia is a common manifestation of COVID-19, and computed tomography (CT) has been explored as a tool to aid diagnosis by constructing artificial intelligence (AI) systems[137-138]. These AI systems have yielded high accuracy for discriminating COVID-19 pneumonia from common pneumonia and predicting the development of critical illness[137]. Additionally, the inclusion of deep-learning features of chest CT findings with clinical data has improved the prognostic accuracy of COVID-19[139]. With chest X-ray imaging being readily available, the development of AI models has been an easily attainable goal. A deep-learning system has been exploited to differentiate between viral and non-viral pneumonia with an area under curve of 0.94~0.98[140]. Furthermore, some studies have incorporated real-time tracking data from mobile phones or wearable sensors to predict and differentiate COVID-19 cases. Integration of symptom monitoring has also helped predict individuals who might develop COVID-19[141]. Finally, AI has also improved the diagnostic accuracy of serological test findings submitted via smartphone application, thus minimizing the reading ambiguity of images[142].
The mechanisms of COVID-19 have sparked research interests in exploring the effects of pharmaceutical interventions and vaccines. While observational studies provide valuable insights into the therapeutic effects of pharmacologic interventions, randomized controlled trials (RCTs) remain the cornerstone of evidence to inform clinical decisions (Fig. 4). In this summary, we primarily focus on the findings from representative randomized controlled trials to provide a comprehensive overview.
The role of viral infections in the pathophysiology of COVID-19 has prompted research into antiviral medications for clinical management. Lopinavir-ritonavir has shown no improvement in major clinical outcome measures, including duration to clinical recovery[143], 28-day all-cause mortality[144], and inhospital mortality[145]. When combined with ribavirin and interferon beta-1a, however, lopinavir-ritonavir has shown the potential to accelerate the negative conversion of viral nucleic acid assays[146]. The novel antiviral drug, nirmatrelvir, when administered with ritonavir, has demonstrated significantly lower viral load and decreased risk of progression to severe COVID-19[147]. Remdesivir has not shown improvement in clinical status[148-149] or reduction in in-hospital mortality among patients with severe COVID-19[145]. The effectiveness of remdesivir in improving clinical status and accelerating clinical recovery remains controversial[150-151]. The timing of drug administration is crucial in determining the efficacy. Early administration of lopinavir-ritonavir (within 10 days of symptom onset) has shown improved clinical outcomes in a post hoc analysis[152]. Recent findings suggest that administration remdesivir within 5 days of symptom onset can improve the clinical outcomes of COVID-19[153]. In another randomized trial, early administration of molnupiravir significantly decreased the risk of disease progression among non-hospitalized patients[154]. Early treatment with ivermectin among out-patient, however, did not significantly reduce the risk of hospitalization[155].
Prevention is another key aspect of disease management. Hydroxychloroquine, despite its failure to improve multiple clinical outcome metrics, including clinical status[156, 157], negative conversion at 28 days[158], in-hospital mortality[145] and 28-day mortality[159], has not demonstrated prophylactic effects in reducing the likelihood of laboratory-confirmed COVID-19[160-161] or COVID-19-compatible illnesses[160]. Results regarding favipiravir have been mixed[162-163], with one study (N = 380) showing no favorable clinical outcomes compared to lopinavir-ritonavir treatment[163]. Ivermectin has not been recommended to shorten the duration to clinical improvement in patients with mild-to-moderate COVID-19[164]. Findings regarding interleukin beta-1a have also been mixed. Despite its failure to reduce inhospital mortality[145], interferon beta-1α has shown superiority compared to placebo in improving the clinical status[165].
The prominent pulmonary and systemic inflammatory responses in COVID-19 have highlighted the potential role of anti-inflammatory medications. Intravenous dexamethasone has been shown to significantly increase ventilator-free days[166] and reduce 28-day mortality in patients with severe COVID-19[167]. Hydrocortisone has demonstrated a significant increase in organ support-free days[168], although there is a considerable risk of treatment failure on day 21[169]. These findings are consistent with initial observations that demonstrated a survival benefit in patients with critical illness[170]. The Janus kinase-3 inhibitor baricitinib, when combined with remdesivir, has been shown to accelerate clinical recovery compared to remdesivir alone[171]. Fluvoxamine has shown effectiveness in preventing progression to severe COVID-19[172], and inhaled budesonide has decreased the risk of emergency room visits[173]. Results on other anti-inflammatory medications, however, have been negative. The macrolide azithromycin has not shown an acceleration in clinical recovery[174], reduction in hospital admission or death within 28 days[174] or 28-day mortality[175], or reduction in the likelihood of acquiring SARS-CoV-2 infections[176]. Ruxolitinib has failed to accelerate clinical improvement, although the trial may have been underpowered[177]. Furthermore, high-dose vitamin D[178] and colchicine[179] have not been effective in shortening hospital stay.
Full details of antiviral and anti-inflammatory medications in the representative published randomized controlled trials are shown in Table 1.
Drug | Enrollment period | The main countries | No. randomized | Dosing scheme | Control | Primary endpoint | Primary endpoint met | Main findings | Main adverse events |
Lopinavir-ritonavir | Feb 10 - Mar 20, 2020 | China | 127 | Ribavirin 400 mg every 12 h, three doses of 8 million international units of interferon beta-1b on alternate days and Lopinavir 400 mg + ritonavir 100 mg twice daily for 14 days | Lopinavir 400 mg + ritonavir 100 mg twice daily for 14 days | Time to nasopharyngeal swab negative for SARS-CoV-2 | Yes | The combination group had a significantly shorter median time from start of study treatment to negative nasopharyngeal swab | Nausea, diarrhea |
Jan 18 - Feb 3, 2020 | China | 199 | Lopinavir 400 mg + ritonavir 100 mg twice daily for 14 days | Standard care | Time to clinical improvement | No | No difference in the time to clinical improvement, viral loads and mortality at 28 days | Nausea, vomiting, and diarrhea | |
Mar 19 - Jun 29, 2020 | United Kingdom | 5, 040 | Lopinavir 400 mg + ritonavir 100 mg twice daily for 10 days until discharge | Standard care | 28-day all-cause mortality | No | No significant difference in time until discharge alive from hospital or the proportion of patients discharged from hospital alive within 28 days | Not specified | |
Mar - Jul 4, 2020 | 30 countries | 11, 330 (1, 411 in lopinavir group) | Two tablets twice daily for 14 days | The local standard of care | in-hospital mortality | No | Death occurred in 148 of 1, 399 patients receiving lopinavir and in 146 of 1, 372 receiving a control drug (rate ratio, 1.00; 95% CI, 0.79 to 1.25) | Not specified | |
Remdesivir | Feb 21 - Apr 19, 2020 | United States | 1, 062 | Remdesivir 200 mg on day 1, followed by 100 mg daily for up to 9 additional days) | placebo for up to 10 days | Time to clinical recovery | Yes | Patients receiving remdesivir had a significantly shorter median recovery time (10 vs. 15 days) | Not specified |
Mar 15-Apr 18, 2020 | United States, Europe and Asia | 596 | Remdesivir intravenously at 200 mg on day 1 followed by 100 mg/d | Standard care | Clinical status on day 11 on a 7-point ordinal scale | Yes | Patients in the 5-day group had significantly higher odds of a better clinical status distribution | Nausea, hypokalemia, and headache | |
Mar 6 - Mar 26, 2020 | United States and other countries | 397 | Intravenous remdesivir for 5 days | intravenous remdesivir for 10 days | Clinical status on day 14, assessed on a 7-point ordinal scale | No | A clinical improvement of 2 points or more on the ordinal scale occurred in 64% of patients in the 5-day group and in 54% in the 10-day group | Nausea, worsening respiratory failure, elevated alanine aminotransferase level, constipation | |
Feb 6 - Mar 12, 2020 | China | 237 | Remdesivir 200 mg on day 1 followed by 100 mg on days 2-10 in single daily infusions for 10 days | The same volume of placebo infusions for 10 days | Time to a decline of two levels on a six-point ordinal scale of clinical status or discharged from hospital | No | Remdesivir use was not associated with a difference in time to clinical improvement | Constipation, hypoalbuminaemia, hypokalaemia, anaemia, thrombocytopenia, increased total bilirubin | |
Mar 2020 - NA | 30 countries | 11, 330 (2, 750 in remdesivir group) | 200 mg on day 0 and 100 mg intravenously on days 1 through 9 | The local standard of care | in-hospital mortality | No | Death occurred in 301 of 2, 743 patients receiving remdesivir and in 303 of 2, 708 receiving a control agent (rate ratio, 0.95; 95% CI, 0.81 to 1.11) | Not specified | |
Hydroxychloroquine | Mar 25 - Jun 5, 2020 | United Kingdom | 4, 716 | The usual standard of care plus hydroxychloroquine or one of the other available treatments | Usual standard of care | 28-day mortality | No | No significant difference in death within 28 days (27.0% in the hydroxychloroquine group vs. 25.0% in the usual-care group) | Supraventricular tachycardia, atrial flutter |
Mar 29 - May 17, 2020 | Brazil | 667 | Standard care plus hydroxychloroquine at 400 mg twice daily plus azithromycin at 500 mg once daily for 7 days | Standard care; standard care plus hydroxychloroquine at 400 mg twice daily | Clinical status at 15 days as assessed with the use of seven-level ordinal scale | No | The odds of having a higher score at 15 days was not affected by hydroxychloroquine alone (odds ratio: 1.21) or hydroxychloroquine plus azithromycin (odds ratio: 0.99) | Prolongation of the QTc interval, elevated liver enzymes | |
Apr 2 - Jul 17, 2020 | United States | 479 | Hydroxychloroquine (400 mg twice daily for 2 doses, then 200 mg twice daily for 8 doses) | Placebo | Clinical status 14 days after randomization (7-category ordinal scale) | No | Clinical status at 14 days did not significantly differ between the hydroxychloroquine and the placebo groups (median: 6 vs. 6). | QTc interval greater than 500 ms | |
Mar - Jun 19, 2020 | 30 countries | 11, 330 (954 in hydroxychloroquine group) | Four tablets at hour 0, four tablets at hour 6, and starting at hour 12, two tablets twice daily for 10 days | The local standard of care | In-hospital mortality | No | Death occurred in 104 of 947 patients receiving hydroxychloroquine and in 84 of 906 receiving a control agent (rate ratio, 1.19; 95% CI, 0.89 to 1.59) | Not specified | |
Feb 11- Feb 29, 2020 | China | 150 | A loading dose of 1, 200 mg daily for three days followed by a maintenance dose of 800 mg daily (total duration: two or three weeks) | Standard care | Negative conversion by 28 days | No | The probability of negative conversion by 28 days in the standard of care plus hydroxychloroquine group (85.4%%) was similar to that in the standard care group (81.3%) | Diarrhoea being most common; serious adverse events rare | |
Mar 17 - May 6, 2020 | United States and Canada | 821 | Hydroxychloroquine 800 mg once, followed by 600 mg in 6-8 hours, then 600 mg daily for 4 additional days | Placebo | Incidence of lab confirmed Covid-19 or illness compatible with Covid-19 within 14 days | No | The incidence of new illness compatible with Covid-19 did not differ significantly | Nausea, loose stools, abdominal discomfort | |
Mar 17 - Apr 28, 2020 | Spain | 2, 314 | Hydroxychloroquine 800 mg once, followed by 400 mg daily for 6 days | Usual care (no specific therapy) | PCR-confirmed, symptomatic Covid-19 within 14 days | No | Similar incidence of PCR-confirmed, symptomatic Covid-19 in the hydroxychloroquine and usual-care groups (5.7% and 6.2%) | Diarrhea, nausea, abdominal pain, drowsiness, headache | |
Ivermectin | Jul 15 - Dec 21, 2020 | Colombia | 476 | ivermectin 300 μg/kg of body weight per day for 5 days | Placebo for 5 days | Time to resolution of symptoms within 21-day follow-up | No | The median time to resolution of symptoms was 10 days in the ivermectin group compared with 12 days in the placebo group | Headache, dizziness, diarrhea, nausea |
Favipiravir | Feb 4 - Mar 8, 2020 | Iran | 380 | Favipiravir (1.6 gr loading, 1.8 gr daily) | Lopinavir/Ritonavir (800/200 mg daily) | The number of admissions to the intensive care unit | No | The number of deaths, intubations, and ICU admissions were not significantly different | Nausea, anorexia, fatigue, headache |
Interferon beta-1a | Mar 30 - May 30, 2020 | United Kingdom | 101 | 6 MIU interferon beta-1a by inhalation via a mouthpiece daily for 14 days | Placebo | Change in clinical condition on the WHO Ordinal Scale for Clinical Improvement (OSCI) during the dosing period | Yes | Patients receiving SNG001 had greater odds of improvement on the OSCI scale (odds ratio 2.32) on day 15 or 16 and were more likely than those receiving placebo to recover to an OSCI score of 1 (no limitation of activities) during treatment | Headache; few serious adverse events |
Mar - Jul 4, 2020 | 30 countries | 11, 330 (2, 063 in interferon beta-1a group) | Subcutaneous: three doses over 6 days (randomization and days 3 and 6) of 44 μg of interferon beta-1a Intravenous: 10 μg daily for 6 days |
The local standard of care | in-hospital mortality | No | Death occurred in 243 of 2, 050 patients receiving interferon beta-1a and in 216 of 2, 050 receiving its control (rate ratio, 1.16; 95% CI, 0.96 to 1.39) | Not specified | |
Corticosteroids | Mar 19 - Jun 8, 2020 | United Kingdom | 2, 104 | Oral or intravenous dexamethasone (6 mg once daily) for up to 10 days | Usual care | 28-day mortality | Yes | Significantly lower percentage of patients died in the dexamethasone group than in the usual care group (22.9% vs. 25.7%) | Not specified |
Apr 17 - Jun 23, 2020 | Brazil | 299 | Dexamethasone intravenously daily for 5 days, 10 mg dexamethasone daily for 5 days or until ICU discharge, plus standard care | Standard care alone | Ventilator-free days during the first 28 days | Yes | Patients in the dexamethasone group had a mean of 6.6 ventilator-free days vs 4.0 ventilator-free days in the standard care group | Insulin use for glycemia | |
Mar 9 - Jun 17, 2020 | Australia and other countries | 614 | A fixed 7-day course of intravenous hydrocortisone (50 mg or 100 mg every 6 hours) A shock-dependent course (50 mg every 6 hours) | No hydrocortisone | Organ support-free days within 21 days (Bayesian cumulative logistic model) | Yes | The adjusted Bayesian probability of superiority was 93% for the fixed-dose hydrocortisone group | Not specified | |
Mar 7-Jun 1, 202 | France | 149 | Continuous intravenous infusion of hydrocortisone at 200 mg/d at day 1 and continued at 200 mg/d until day 7 and then decreased to 100 mg/d for 4 days and 50 mg/d for 3 days | Placebo (saline) for 14 days | Treatment failure on day 21 | No | No significant difference in the percentage of patients (42.1%) in the hydrocortisone group and in the placebo group (50.7%), who reached the primary endpoint | Not specified | |
Azithromycin | Apr 7 - Nov 27, 2020 | United Kingdom | 16, 442 | Usual standard of care plus azithromycin 500 mg once per day by mouth or intravenously for 10 days or until discharge | Usual standard of care alone for 10 days or until discharge | 28-day all-cause mortality | No | No significant difference in the 28-day all-cause mortality between the azithromycin group and the control group (22% vs. 22%) | Not specified |
May 22 - Nov 30, 2020 | United Kingdom | 2, 265 | Usual care plus azithromycin 500 mg daily for three days | Usual care plus other interventions, or usual care alone | Time to first recovery in 28 days Hospital admission or death related to COVID-19 in 28 days |
No | No significant effect by azithromycin in time to first reported recovery; No significant difference in the percentage of participants being hospitalized or died | Not specified | |
Mar 28 - May 19, 2020 | Brazil | 447 | Azithromycin (500 mg via oral, asogastric, or intravenous administration once daily for 10 days) plus standard of care | Standard of care without macrolides | SARS-CoV-2 infection confirmed by testing before randomisation | No | SARS-CoV-2 infection was not significantly different between the azithromycin and control groups (odds ratio: 1.36) | QTc interval prolongation, gastrointestinal intolerance | |
Baricitinib | May 8- Jul 1, 2020 | United States | 1, 033 | Remdesivir: 200-mg on day 1, 100-mg daily on days 2-10 or until hospital discharge or death Baricitinib: 4-mg daily dose for 14 days or until hospital discharge |
Placebo | Time to recovery within 15 days | Yes | Patients receiving baricitinib experienced a shorter time to recovery and had higher odds of improvement in clinical status at day 15 | Hyperglycemia, anemia, decreased lymphocyte count, acute kidney injury |
Fluvoxamine | Apr 10 - Aug 5, 2020 | United States | 181 | 100 mg of fluvoxamine 3 times daily for 15 days | Placebo 3 times daily for 15 days | Clinical deterioration within 15 days of randomization | Yes | Clinical deterioration occurred in 0 of 80 patients in the fluvoxamine group and in 6 of 72 patients in the placebo group | Pneumonia, nausea, vomiting |
Inhaled budesonide | Jul 16 - Dec 9, 2020 | United Kingdom | 146 | Budesonide dry powder 800 μg twice daily until symptom resolution | Usual care | COVID-19-related urgent care visit | Yes | The primary outcome occurred in 15% participants in the usual care group and 3% in the budesonide group | Sore throat; dizziness |
Ruxolitinib | Feb 9 - Feb 28, 2020 | China | 43 | Ruxolitinib 5 mg twice a day plus standard-of-care treatment | Placebo (100 mg vitamin C) twice daily with standard care | Time to clinical improvement | No | No differences in terms of clinical improvement between ruxolitinib (median: 12 days) and placebo group (median: 15 days) | Anemia, thrombocytopenia, hypercholestrolemia |
Vitamin D | Jun 2 - Aug 27, 2020 | Brazil | 240 | A single oral dose of 200 000 IU of vitamin D3 | A single dose of placebo | Length of stay | No | Median length of stay was not significantly different between the vitamin D3 (7.0 days) and placebo groups (7.0 days) | Not specified |
The robust inflammatory responses observed in patients with severe or critical illness, particularly the cytokine release syndrome, have prompted investigations into the efficacy of biologics targeting interleukin-1β, interleukin-6, and granulocyte colony stimulating factor (G-CSF). The REMAP-CAP trial reported a markedly longer duration free from respiratory and cardiovascular support with both tocilizumab and sarilumab[180], while the RECOVERY trial demonstrated a significant reduction in 28-day mortality among patients with severe and critical illness treated with tocilizumab[181]. However, other clinical trials have shown no benefits of tocilizumab in improving the clinical status[182-184], disease progression[185], intubation or mortality[186-187]. Similarly, sarilumab failed to shorten the time to clinical improvement[188]. Marilimumab did not increase the proportion of patients alive and weaning from oxygen therapy[189], but G-CSF supplementation effectively reduced the risk of progression to critical illness or death in patients with profound lymphopenia[190]. Anakinra, an interleukin-1 antagonist, was also ineffective in reducing the risk of invasive ventilation or death[191]. Treatment with vilobelimab, a complement 5 antagonist, did not effectively improve oxygenation compared to best supportive care[192]. Therefore, precise intervention is necessary to maximize the benefit of biologics treatment. For detailed information on biologics, please refer to Table 2, which provides an overview of representative published randomized controlled trials.
Drug | Enrollment period | The main countries | No. randomized | Dosing scheme | Control | Primary endpoint | Primary endpoint met | Main findings | Main adverse events |
Tocilizumab | Mar 9 - Nov 19, 2020 | United Kingdom | 755 | Tocilizumab (8 mg per kilogram of body weight) | Standard care alone | Respiratory and cardiovascular organ support-free days to day 21 | Yes | The median number of organ support-free days was 10 in tocilizumab group and 0 in control group (median adjusted cumulative odds ratios: 1.64) | Not specified |
Apr 23, 2020, - Jan 24, 2021 | United Kingdom | 4, 116 | Tocilizumab 400 mg-800 mg given intravenously (the 2nd dose can be given 12-24 h later) plus standard of care | Standard of care alone | 28-day mortality | Yes | 31% of patients allocated tocilizumab and 35% of patients allocated to usual care died within 28 days (rate ratio 0·85; 95% CI 0·76-0·94) | Not specified | |
Apr 20 - Jun 15, 2020 | United States | 243 | Tocilizumab (8 mg per kilogram of body weight administered intravenously, not to exceed 800 mg) | Placebo | Intubation or death, assessed in a time-to-event analysis | No | The hazard ratio for intubation or death in the tocilizumab group compared with the placebo group was 0.83 (95% confidence interval: 0.38 - 1.81) | Neutropenia, elevated liver enzyme | |
Apr 3 - May 28, 2020 | Europe and North America | 452 | A single intravenous infusion of tocilizumab (at a dose of 8 mg per kilogram of body weight) | Placebo intravenous injection | Clinical status at day 28 on an ordinal scale ranging from 1 to 7 | No | The median value for clinical status at day 28 was 1.0 in the tocilizumab group and 2.0 in the placebo group | Infection, bleeding, hypersensitivity | |
May 30 - Aug 31, 2020 | India | 180 | Tocilizumab 6 mg/kg plus standard care | Standard care alone | Progression of COVID-19 up to day 14 | No | Progression of COVID-19 occurred in 9% of patients in the tocilizumab group and 13% in the standard care group (difference −3·71; p=0·42) | Infections | |
Mar 31 - Apr 18, 2020 | France | 131 | Tocilizumb 8 mg/kg, intravenously plus usual care on day 1 and on day 3 if clinically indicated | Usual care alone | Scores higher than 5 on WHO 10-point Clinical Progression Scale on day 4 and survival without ventilation at day 14 | No | In the tocilizumab group, 12 patients vs 19 in the usual care group had scores higher than 5. At day 14, 12% fewer patients needed ventilation or died in the tocilizumab group. | Hepatic cytolysis, anemia, neutropenia | |
Mar 31 - Jun 11, 2020 | Italy | 126 | Intravenous tocilizumab within 8 hours from randomization (8 mg/kg up to 800 mg), followed by a second dose after 12 hours | Supportive care until clinical worsening (could receive tocilizumab) | Entry into intensive care unit with invasive ventilation, death from all causes, or clinical aggravation | No | 28.3% in tocilizumab arm and 27.0% in the standard care group showed clinical worsening within 14 days. Two patients in the tocilzumab group and 1 in the control group died before 30 days; 6 and 5 patients were intubated | Laboratory abnormalities, infection, infestation | |
May 8- Jul 20, 2020 | Brazil | 129 | Tocilizumab (single intravenous infusion of 8 mg/kg) plus standard care | standard care alone | Clinical status measured at 15 days | No | 28% of patients in the tocilizumab group and 20% in the standard care group were receiving mechanical ventilation or died at day 15 (odds ratio, 1.54, P=0.32) | Abnormal liver function, anemia, thrombocytopenia | |
Sarilumab | Jun 20 - Nov 19, 2020 | United Kingdom | 755 | Sarilumab (400 mg) | Standard care alone | Respiratory and cardiovascular organ support-free days up to day 21 | Yes | The median of organ support-free days was 11 in the sarilumab group and 0 in the control group | Not specified |
Mar 28 - Jul 3, 2020 | Argentina and other countries | 431 | intravenous sarilumab 400 mg, sarilumab 200 mg | Placebo | Time to clinical improvement of two or more points (seven point scale) | No | No significant differences in median time to improvement between the placebo (12.0 days) and sarilumab 200 mg (10.0 days) or 400 mg groups (10.0 days) | Increased alanine aminotransferase, invasive bacterial or fungal infection | |
Anakinra | Apr 8- Apr 26, 2020 | France | 116 | Usual care plus anakinra (200 mg twice daily on days 1-3, 100 mg twice on day 4, 100 mg once on day 5) | Usual care alone | Proportion of patients who had died or needed ventilation by day 4; Survival without ventilation at day 14 | No | 36% of patients in the anakinra group had WHO-CPS score > 5 at day 4 versus 38% in the usual care group; 47% patients in the anakinra group and 51% in the usual care group needed ventilation or died |
Acute respiratory distress syndrome, bacterial sepsis, hepatic cytolysis |
Granulocyte colony stimulating factor | Feb 18 - Apr 10, 2020 | China | 199 | 3 doses of recombinant human granulocyte colony-stimulating factor (5 μg/kg, subcutaneously at days 0-2) | Standard care | The time to improvement of at least 1 point on 7-category severity score | No | Time to clinical improvement was similar (median 12 days in the rhG-CSF group vs 13 days in the usual care group; hazard ratio, 1.28; 95% CI, 0.95-1.71) | Neutrophilia, osteodynia, muscle soreness |
Marilimumab | May 28 - Sep 15, 2020 | United States | 40 | Mavrilimumab 6 mg/kg as a single intravenous infusion | Placebo intravenous infusion | The proportion of patients alive and off supplemental oxygen therapy at day 14 | No | Similar percentages of patients (odds Ratio: 1·48, 95% confidence interval: 0·43-5·16) remained alive and were off supplemental oxygen therapy | Bacterial pneumonia |
Vilobelimab | Mar 31 - Apr 24, 2020 | The Netherlands | 30 | Vilobelimab (up to seven doses of 800 mg intravenously) plus best supportive care | Best supportive care | Percentage change in PaO2/FiO2 in supine position between baseline and day 5 | No | No differences between treatment groups (17% change in the vilobelimab group vs 41% in the control group; difference 24% [95% CI: 58 to 9]). | pulmonary embolisms; infections |
Drawing inspiration from the successful immunization using convalescence plasma among individuals infected with SARS[193], clinical trials have been conducted to evaluate the effects of convalescence plasma in patients with COVID-19. While high titers of convalescent plasma and monoclonal antibodies[194] have shown accelerated reduction in viral loads[195-197], the findings from published RCTs have been inconsistent[198-202]. Meta-analysis of these trials revealed no overall benefit in terms of reducing mortality, length of hospital stay, and use of mechanical ventilation[203]. It is important to note that previous trials may have been limited by delayed administration, which could contribute to the negative results. However, a recent trial showed that administration convalescent plasma within 9 days of symptom onset among unvaccinated patients significantly reduced the risk of disease progression leading to hospitalization[204].
The involvement of ACE2 in facilitating viral entry has raised concerns about the use of ACE inhibitors among COVID-19 patients. Encouragingly, both ACE inhibitors and angiotensin-receptor blockers have been found to be unrelated to the risk of contracting SARS-CoV-2 infections[205-208] or progressing to critical illnesses or death[209]. Furthermore, discontinuation of ACE inhibitors and angiotensin-receptor blockers did not impact the number of days alive[210], indicating that these treatments can be safely continued in patients with cardiovascular comorbidities[211].
To enhance the fight against COVID-19, vaccines targeting various structural components of SARS-CoV-2 have been developed, including inactivated vaccine, recombinant S-protein vaccine, chimpanzee adenovirus vaccine, and mRNA vaccine. Robust immunogenicity has been observed with two doses of these vaccines, while a single dose typically elicits modest immune response[77, 86, 89, 212-236]. However, markedly lower among cancer patients, immunogenicity was significantly lower after a single dose but could be improved with a booster dose[223]. Two-dose vaccine has been reported to range from 66.7% to 95% in the general population[77, 216, 218-220], 230-231], except for the lower efficacy of chimpanzee adenovirus vaccine[89]. Real-world data has shown notable vaccine efficacy across all age groups in preventing COVID-19[212]. Adverse events were generally mild and included injection-site pain, fever, and myalgia. Cases of thromboembolism or thrombocytopenia associated with antibodies against platelet factor-4 have been reported following the administration of chimpanzee adenovirus vaccine[237-239] and adenovirus 26 vaccines[240]. The burden of thromboembolism or thrombocytopenia is considerable, with a standardized morbidity ratio of 2.5 (95% confidence interval, 0.97 to 2.25)[241]. More information about vaccines reported in RCTs are summarized in Table 3. Waning immunity, occurring 2-6 months after two-dose vaccination, along with the emergence of new variants, has contributed to the ongoing increase in COVID-19 cases globally[242-243]. These observations highlight the need for global vaccination efforts. Choosing the best vaccination strategy in light of the availability of different vaccines is an important question. Both homologous and heterologous vaccination strategies have demonstrated significantly increased neutralizing antibody titers[244]. Pooled analyses from randomized controlled trials have shown the superior immunogenicity of heterologous compared to homologous vaccination in the general population[245-246] and the elderly[247]. Non-inferiority has been established when comparing different approaches to implementing heterologous booster vaccination[248]. Further boosters, such as a fourth shot, may not provide significant additional benefits compared to a third-dose booster[95].
Vaccine | Enrollment period | The main countries | Participants | No. randomized | Dosing scheme | Control | Primary endpoint | Vaccine efficacy | Efficacy against variants | Main immunogenicity outcomes | Main safety outcomes |
mRNA-1273 | Apr 16 - May 12, 2020 | United States | Older adults | 40 | Two doses of either 25 μg or 100 μg of vaccine administered 28 days apart | NA | Antibody responses on days 1, 15, 29, 36, 43, and 57 | NA | NA | Anti-S-2P GMT: 25-μg: 323, 945 in 56-70 yr; 1, 128, 391 in ≥71 yr group; 100-μg: 1, 183, 066 and 3, 638, 522 | Predominantly mild or moderate in severity (fatigue, chills, headache, myalgia, pain) |
Jul 27 - Oct 23, 2020 | United States | Individuals 18 years of age or older | 30, 420 | A two-dose regimen containing 0.5 mL injections containing 100 μg of mRNA-1273 | Placebo (saline) | Prevention of Covid-19 with onset > 14 days after 2nd injection in non-infected participants | 94.1% | NA | NA | (Mainly) pain after injection | |
BNT162b1 | May 4 - Jun 22, 2020 | United States | Healthy adults (18-55 yrs; 65-85 yrs) | 195 | Two doses of vaccine (10 μg, 20 μg, 30 μg, and 100 μg), with a 21-day interval; One dose 100 μg of BNT162b1 in one group only | Placebo | Safety ( e.g. , local and systemic reactions and adverse events) | NA | NA | Similar dose-dependent neutralizing GMTs in younger and older adults | Lower incidence and severity of systemic reactions than BNT162b2 |
BNT162b2 | Jul 27, - Nov 14, 2020 | United States and other countries | Adults 16 yrs or older | 43, 548 | BNT162b2 vaccine twice (30 μg per dose), at 21-day interval | Placebo | Efficacy against laboratory-confirmed Covid-19 and safety | 95% | NA | NA | Short-term, mild-to-moderate pain at injection site, fatigue, headache |
Dec 20, 2020, to Feb 1, 2021 | Israel | Non-infected individuals aged 16 yrs or greater | 596, 618 | Having received BNT162b2 vaccination | No vaccination | COVID-19 infection, symptomatic Covid-19, hospitalization, severe illness, death | 7 days after 2nd dose: 92% | NA | NA | NA | |
Dec 8 - Dec 29, 2020 | United Kingdom | Patients with cancer | 151 | Two 30 μg doses of BNT162b2 administered intramuscularly 21 days apart | Single dose of vaccine | Seroconversion to spike protein and vaccine boosting after 21 days on seroconversion | NA | NA | One dose yields poor efficacy. Immunogenicity increased in patients with solid cancer in 2 weeks of boost | No toxicities in 54% patients following the first dose | |
Ad26.COV2.S | Jul 22 - Aug 7, 2020 | Belgium and the United States | Healthy adults aged 18-55 yrs and 65 yrs or greater | 805 | 5×1010 viral particles (low dose); 1×1011 viral particles (high dose) per milliliter |
Placebo | Safety and reactogenicity | NA | NA | Neutralizing antibody detected in ≥90% of participants on day 29 after dose 1 and was 100% by day 57 | NA |
Sep 21, 2020 - Jan 22, 2021 | Argentina, Brazil and other countries | Adults 18-59 yrs and 60 yrs or older | 19, 630 | 5×1010 viral particles as a single intramuscular injection (0.5 mL) | Placebo | Vaccine efficacy against moderate to severe-critical Covid-19 with an onset at least 14 and 28 days | 76.7% at ≥14 days; 85.4% at ≥28 days | 52.0% and 64.0% (20H/501Y. V2 variant) | Higher reactogenicity than with placebo (mild to moderate and transient) | injection-site pain, headache, fatigue, myalgia, nausea | |
Jun 18 - Aug 3, 2020 | Russia | Adults aged 18-60 yrs | 76 | One dose of rAd26-S or one dose of rAd5-S | NA | SARS-CoV-2-specific antibodies on days 0, 14, 21, 28, and 42; number of participants with adverse events | NA | NA | At day 42, RBD IgG titers were 14703 (frozen) and 11 143 (lyophilised) Neutralising antibodies: 49·25 and 45·95, respectively | Common: pain at injection site, hyperthermia, headache, asthenia, muscle and joint pain | |
Jul 29 - Aug 7, 2020 | United States | Participants 18-55 yrs old | 25 | 1 or 2 intramuscular injections with 5 × 1010 viral particles or 1 × 1011 viral particles of Ad26. COV2.S vaccine | Placebo | Cellular immune responses and T cell responses | NA | NA | Binding and neutralizing antibodies emerged by day 8 in 90% and 25% of vaccine recipients | NA | |
Recombinant spike protein nanoparticle vaccine | May 26, - Jun 6, 2020 | Australia | Young and middle-aged adults | 131 | rSARS-CoV-2 vaccine (in 5-μg and 25-μg doses, with or without Matrix-M1 adjuvant) | Placebo | Reactogenicity; serum chemistry, hematology; IgG anti-spike protein response | NA | NA | Two-dose 5-μg regimen induced marked anti-spike IgG and neutralization responses | No serious adverse events; Overall reactogenicity was absent or mild |
Aug 17 - Nov 25, 2020 | South Africa | HIV-positive and -negative adults aged 18-64 yrs | 4, 387 | NVX-CoV2373 (5 μg of recombinant spike protein with 50 μg of Matrix-M1 adjuvant) | Placebo (0.5 mL) | Safety and vaccine efficacy against lab-confirmed symptomatic Covid-19 at 7 days or more after 2nd dose | 60.1% | 51.0% | Vaccine efficacy among HIV-negative participants was 60.1% | Headache, muscle pain, and fatigue most common; no serious adverse events | |
Recombinant spike protein vaccine | Sep 3 - Sep 29, 2020 | Untied States | Adults 18-49 yrs and ≥50 yrs | 441 | One dose (on day 1) or two doses (on days 1 and 22) of placebo or candidate vaccine, containing low dose (effective dose 1·3 μg) or high-dose (2·6 μg) antigen with adjuvant AF03 or AS03 | Unadjuvanted high-dose antigen | Safety up to day 43, and immunogenicity (neutralising antibodies on days 1, 22, and 36) | NA | NA | Neutralizing antibody titers: 13·1 in low-dose + AF03 group, 20·5 in low-dose + AS03 group, 43·2 in high-dose + AF03 group, 75·1 in high-dose + AS03 group | No vaccine-related unsolicited immediate adverse events, serious adverse events |
ChAdOx1 nCoV-19 vaccine | Jun 24 - Nov 9, 2020 | South Africa | Adults 18-65 yrs | 2, 026 | Two doses of vaccine containing 5×1010 viral particles 21 to 35 days apart | Placebo (0.9% saline) | Safety and efficacy against lab-confirmed symptomatic Covid-19 > 14 days after 2nd dose | 21.9% | 21.9% | Strong neutralizing antibodies at 28 days after dose 1 and increased after dose 2 | No serious adverse events |
Apr 23 - May 21, 020 | United Kingdom | Healthy adults aged 18-55 years | 1, 077 | ChAdOx1 nCoV-19 vaccine (5×1010 viral particles) | Menigococcal ACWY vaccine | Cases of symptomatic virologically confirmed COVID-19; Occurrence of serious adverse events |
NA | NA | Anti-spike IgG responses rose by day 28, and were boosted following a second dose | Pain, feverish, chills, muscle ache, headache, malaise; No serious adverse events |
|
May 30 - Aug 8, 2020 | United Kingdom | Adults aged 18-55 yrs, 56-69 yrs, and 70 yrs and older | 560 | intramuscular ChAdOx1 nCoV-19 (2.2×1010 virus particles) | Meningococcal ACWY vaccine | The number of cases of symptomatic, virologically confirmed COVID-19, serious adverse events | NA | NA | Median anti-spike IgG responses 28 days after the boost dose were similar across the three age cohorts | Injection-site pain, feeling feverish, muscle ache, headache (less common in older adults) | |
Apr 23 - Nov 4, 2020 | United Kingdom, Brazil, South Africa | Adults aged 18 yrs and older | 23, 848 | Two doses containing 5×1010 viral particles; A half dose as their first dose; A standard dose as their second dose |
Meningococcal ACWY vaccine | Symptomatic COVID-19 in seronegative participants with PCR-positive swab > 14 days after a second dose | 70.4% | NA | Two standard doses: 62.1%; Low dose followed by standard dose: 90.0% |
Good safety profile with serious adverse events balanced across the study arms | |
Apr 23 - Dec 6, 2020 | United Kingdom, Brazil | individuals aged 18 years and older | 24, 422 | Two standard doses of ChAdOx1 nCoV-19 (5×1010 viral particles) | Control vaccine or placebo | Virologically confirmed symptomatic COVID-19 disease | 66.7% | NA | Antibody levels were maintained with minimal waning by day 90 (geometric mean ratio 0·66 [95%CI 0·59-0·74]) | The most common serious adverse events were infections and infestations | |
May 31 - Dec 30, 2020 | United Kingdom | Healthy people aged 18 years and older | 8, 534 | Standard-dose ChAdOx1 nCoV-19 vaccine (5×1010 viral particles) | Meningococcal conjugate control vaccine | Symptomatic COVID-19 in seronegative participants with positive swab > 14 days after dose 2 | 81.5% for non-B.1.1.7 variant | 70.4% for B.1.1.7 variant | NA | NA | |
BBIBP-CorV | Phase 1: Apr 29 - Jun 28, 2020 Phase 2: May 18 - Jul 30, 2020 |
China | Healthy people Phase 1: 18-80 yrs Phase 2: 18-59 yrs |
640 | A single-dose schedule of 8 μg on day 0; A two-dose schedule of 4 μg on days 0 and 14, 0 and 21, or 0 and 28 |
Placebo | Safety and tolerability | NA | NA | Neutralizing antibody titers on day 28 were greater in the 4 μg days 0 and 14, days 0 and 21, and days 0 and 28 schedules | Mild or moderate; No serious adverse event within 28 days |
Inactivated vaccine | Apr 16 - Apr 25, 020 | China | Adults aged 18-59 years | Phase 1: 144 Phase 2: 743 |
Low dose CoronaVac [3 μg per 0·5 mL of aluminium hydroxide diluent per dose]; High-dose Coronavc [6 μg per 0·5 mL of aluminium hydroxide diluent per dse] |
Placebo | Adverse reactions within 28 days; Seroconversion rates of neutralizing antibodies at day 14 and 28 | NA | NA | Phase 1: Seroconversion rates: 46% in 3μg group, 50% in 6μg group, 0% in placebo group Phase 2: 92%, 98% and 3% |
Phase 1: 29% in the 3μg group, 38% in 6μg group, 8% in the placebo group Phase 2: 33%, 35% and 22% |
July 13 - Jul 30, 2020 | United Kingdom and Brazil | Healthy adults aged 18-55 years | 827 | 3 μg with Algel-IMDG, 6 μg with Algel-IMDG, or 6 μg with Algel | Algel only | Solicited local and systemic reactogenicity events at 2 h and 7 days and throughout the study | NA | NA | Seroconversion rates (%) were 87·9, 91·9, and 82·8 in the 3 μg with Algel-IMDG, 6 μg with Algel-IMDG, and 6 μg with Algel groups | Mild or moderate; more frequent after the first dose | |
May 22 - Jun 1, 2020 | China | healthy adults aged 60 yrs and older | 72 | CoronaVac at 1·5 μg, 3 μg, or 6 μg per dose | Placebo | Adverse reactions within 28; seroconversion rate at 28 days after the second injection | NA | NA | Seroconversion was seen in 90.7% of participants in the 1·5 μg group, 98% in the 3 μg group and 99·0% in the 6 μg group | Mild or moderate in severity; injection site pain was most frequent | |
April 12 - Jul 27, 2020 | China | Healthy adults aged 18-59 yers | Phase 1: 96 Phase 2: 224 |
2.5, 5, and 10 μg/dose inactivated vaccine | Aluminum hydroxide adjuvant | Combined adverse reactions at 7 days Neutralizing antibody response after 14 days | NA | NA | GMTs of neutralizing antibodies in low-, medium-, and high-dose groups at day 14: 316, 206 and 297 in phase 1 | Injection site pain, followed by fever (mild and self-limiting) | |
Non-replicating adenovirus type-5-vectored COVID-19 vaccine | Apr 11 - 16, 2020 | China | Healthy adults aged 18 yrs or older | 603 | 1×1011 viral particles per mL or 5×1010 viral particles per mL | Placebo | GMTs of specific ELISA antibody responses to RBD and neutralising antibody responses at day 28 | NA | NA | The RBD-specific ELISA antibodies peaked at 656·5 and 571·0, with seroconversion rates at 96% and 97% at day 28 | Mostly mild to moderate adverse events; pain being the most common adverse event |
Jun 19 - Sep 23, 2020 | Australia | Younger (18-54 yrs) and older adults (55-75 yrs) | 151 | S-trimer vaccine either 3 μg, 9 μg, or 30 μg | Placebo (0.9% saline) | Safety, tolerability, and immunogenicity of three increasing doses | NA | NA | Fixed doses vaccine induced high titers and seroconversion rates of binding and neutralizing antibodies | Well tolerated overall | |
MF59-adjuvanted subunit vaccine | Jun 23 - Aug 17, 2020 | Australia | Healthy adults (aged ≥18 to ≤55 years) | 314 | Two doses via intramuscular injection 28 days apart of either sclamp vaccine at 5 μg, 15 μg, or 45 μg, or one dose of sclamp vaccine at 45 μg followed by placebo | Placebo | Antigen-specific IgG titer at 28 days; Solicited adverse events in the 7 days Unsolicited events up to 12 months |
NA | NA | Vaccination with SARS-CoV-2 clamp elicited a similar antigen-specific response irrespective of dose | Severe solicited reactions occurred at similar rates in participants receiving vaccines at any dose |
GMT: geometric mean titer; RBD: receptor-binding domain; NAAT: nucleic acid amplification test |
Several issues regarding vaccination still need to be addressed. Firstly, it is important to note that vaccine efficacy has been determined based on individual clinical trials conducted in various settings. Therefore, claiming direct superiority of mRNA vaccine efficacy over Ad26.COV2_S vaccine based on a simple comparison is not accurate. Secondly, the antibody responses elicited by mRNA-1273 vaccines have shown a protective effect that lasts for at least 119 days[249] and potentially up to 202 days[250]. However, the true durability of these responses remains unclear due to variations in statistical models used for estimation. Thirdly, there are challenges to overcome in promoting mass vaccination. Achieving sufficient coverage among the elderly population is crucial for establishing herd immunity. Finally, the primary goal of vaccination is to prevent hospitalization and the progression to severe or critical illness, including death. Reassuringly, all vaccines have demonstrated effectiveness in meeting this expectation.
Few published studies have examined the role of mechanical ventilation in patients with COVID-19[251], likely due to concerns about airborne transmission to healthcare workers[252]. In comparison to nasal high-flow oxygen therapy, helmet non-invasive ventilation did not s lead to a reduction in ventilator support days or mortality within 28 days. However, it significantly decreased the likelihood of requiring endotracheal ventilation in hypoxemic patients with mild-to-moderate COVID-19[253]. Outside the intensive care unit, non-invasive ventilation proved beneficial by reducing the respiratory rate, improving oxygenation[254], and lowering mortality rate[252]. Prone positioning has shown to rapidly improve oxygen desaturation in approximately 50% of patients when transitioning back to the supine position[255]. The feasibility of invasive ventilation has also been established recently, with age, gender, tidal volume, respiratory compliance, heart rate, and arterial pH value identified as major predictors of 28-day mortality[256].
Despite the initial studies reporting high mortality rates, efforts have been undertaken to assess the potential benefits of extracorporeal membrane oxygenation (ECMO) for critically ill patients. Encouraging findings emerged from the Extracorporeal Life Support Organization Registry study, which reported a 90-day mortality of 39%[257]. A French study reported a 60-day mortality rate of 36% and a high incidence (42%) of major bleeding[258]. The high mortality rate observed could be attributed to factors such as advanced age, prolonged intubation and ECMO, renal failure, and importantly, the level of experience individual hospitals have with ECMO procedures on an annual basis. However, it is important to note that none of these studies were RCTs, limiting the ability to fully confirm the therapeutic benefits of ECMO.
Lung transplantation has traditionally been reserved as a life-saving intervention for end-stage chronic lung diseases. In the context of COVID-19, suitable candidates for lung transplantation were individuals experiencing refractory hypoxemia and displaying irreversible pulmonary lesions, such as honeycombing fibrosis, parenchymal necrosis, cavernous changes, which did not respond to the best available treatment within four weeks of the onset of acute respiratory distress syndrome. Despite the technical challenges associated with severe pleural adhesion, patients who underwent lung transplantation at high-volume transplant medical centers were successfully weaned off extracorporeal support, and their short-term survival rates were comparable to those of non-COVID-19 transplant recipients[260].
The impact of COVID-19 on systemic symptoms can extend well beyond convalescence period. Some symptoms, such as new-onset dyspnea, have been identified up to four months after hospital discharge[261]. Fatigue or muscle weakness (63%), sleep difficulties (26%), anxiety and depression (23%) persist for at least six months. While exercise tolerance and diffusing capacity tend to improve over time, they are more significantly affected in patients who experienced severe COVID-19[24]. Reduced diffusing capacity may persist for over a year[261]. Radiologic abnormalities, including interstitial lung disease, are common during the convalescent phase[261, 263]. Approximately 33% of patients developed neurological or psychiatric disorders within six months[264]. Furthermore, around 30% of patients require readmission to the hospital within a median of five months, with readmission and mortality rates being four and eight times higher, respectively, than those of non-COVID-19 controls[265].
These consequences emphasize the need for follow-up care and interventions to expedite recovery. The world e has now entered the era of post-COVID-19 management, especially considering that the number of convalescent cases far exceeds that of newly diagnosed or hospitalized cases. Pulmonary rehabilitation has shown promising results in improving exercise tolerance, lung function, and quality-of-life in patients who have recovered from mild to severe COVID-19[266]. It is important to evaluate other therapeutic interventions that could potentially accelerate recovery and assess the long-term effectiveness of pulmonary rehabilitation.
Currently, the global community continues to face imminent or persistent threats posed by the COVID-19 pandemic. First and foremost, it is crucial for governments to prioritize the normalization of social orders. This includes strengthening vaccination efforts, resuming trade and business activities, and promoting the safe reopening of schools and workplaces.
Secondly, effectively managing the pandemic requires global investment and comprehensive support. WHO plays a vital role in guiding and mediating international healthcare affairs. However, the WHO has faced challenges due to insufficient funding and administrative support, leading to a weakened position. Involvement of politics has further eroded the public trust and the scientific community.
Third, the feasibility and practicality of the goal proposed by Tom Frieden, director of the U.S. Centers for Disease Control and Prevention, that every country should identify and investigate new outbreaks within a specific timeframe remains uncertain[267]. It is essential to determine if this goal can be effectively implemented and acted upon.
Fourth, establishing clear standards for declaring a Public Health Emergency of International Concern (PHEIC) is crucial, as such declarations can significantly impact international trade. Criteria for issuing a PHEIC should include factors such as the emergence of a lethal infectious respiratory disease resulting in infections among healthcare workers or clusters of cases in community settings. However, introducing an intermediate level of PHEIC could potentially complicate the system and cause confusion among the public.
Finally, the prevention of future pandemics hinges on achieving sufficient vaccination coverage worldwide. This is especially critical in underdeveloped countries where there is a shortage of vaccine supply. This is especially critical in underdeveloped countries where there is a shortage of vaccine supply. Ensuring equitable access to vaccines across the globe is essential for successful prevention efforts.
Research on coronavirus has not progressed as rapidly as desired, despite the SARS outbreak. This may be attributed to inadequate awareness of emerging deadly coronaviruses. In order to protect human health, it is crucial to continuously strengthen investment in coronavirus and other respiratory virus research. The scientific community has made it a priority to accelerate translational research efforts. Developing new antiviral treatments that are effective against various variants is of great clinical significance. To this end, it is necessary to determine the best approach for validating a new diagnostic method to identify asymptomatic individuals and effectively prevent the spread of COVID-19 from these individuals. Gaining a deeper understanding of the immune evasion mechanisms by different variants and the developing new vaccines are urgent global tasks. Additionally, assessing the long-term protective effects of vaccines is essential to gauge the risk of infection considering the gradual decline in antibody responses over time. Table 4 provides an overview of the key unanswered questions and future research directions in the battle against COVID-19.
Key unanswered questions related to COVID-19 | Proposed future research directions |
The origin of SARS-CoV-2 and the mode of zoonotic transmissions | To unravel the reservoir of SARS-CoV-2 and understanding the mechanisms of zoonotic infections |
Durability of infectiousness of viral particles in the environment | To investigate the immune escape mechanisms of the emerging variants |
Immunologic mechanisms contributing to the sudden deterioration of clinical status and the clinical recovery | To validate a novel diagnostic method for identifying asymptomatic individuals |
The upper limit of duration of the protective effect of antibodies after natural infection and the receipt of vaccination | To assess the effectiveness of mass vaccination programs in reducing global incidence of COVID-19 |
Factors associated with re-infection | To develop new rapid diagnostic testing for point-of-care use |
Molecular mechanisms underlying prolonged shedding of the live viruses or viral fragments | To identify monoclonal antibody cocktails to improve clinical outcomes |
Association between variants and the efficacy of different types of vaccines | To develop medications that target cellular surface markers in addition to ACE2 |
The mechanisms leading to interstitial lung diseases and the reversibility with immunosuppressants during convalescent phase | To determine the optimal treatment options for non-intubated patients with severe COVID-19 |
The probability and duration of complete recovery after discharge from hospital | To explore new intervention strategies to accelerate the functional and psychological recovery post-discharge from COVID-19 |
Humanity has persevered through the darkest period of the COVID-19 pandemic, marked by a lack of understanding of the virus and a critical shortage of essential supplies and vaccines. However, we have gained valuable knowledge and acquired necessary resources that will undoubtedly contribute to enhanced preparedness for future waves of the pandemic.
[1] |
Koh D, Sng J. Lessons from the past: perspectives on severe acute respiratory syndrome. Asia Pac J Public Health, 2010; 22(3): 132S-136S.
|
[2] |
Donnelly C A, Malik M R, Elkholy A, et al. Worldwide Reduction in MERS Cases and Deaths since 2016. Emerg Infect Dis, 2019; 25(9): 1758-1760. doi: 10.3201/eid2509.190143
|
[3] |
World Health Organization. WHO Coronavirus (COVID-19) Dashboard.
|
[4] |
Zhu N, Zhang D, Wang W, et al. A Novel Coronavirus from patients with pneumonia in China, 2019. N Engl J Med, 2020; 382(8): 727-733. doi: 10.1056/NEJMoa2001017
|
[5] |
Zhang Y Z, Holmes E C. A genomic perspective on the origin and emergence of SARS-CoV-2. Cell, 2020; 181(2): 223-227. doi: 10.1016/j.cell.2020.03.035
|
[6] |
Passamonti F, Cattaneo C, Arcaini L, et al. Clinical characteristics and risk factors associated with COVID-19 severity in patients with haematological malignancies in Italy: a retrospective, multicentre, cohort study. Lancet Haematol, 2020; 7(10): e737-e745. doi: 10.1016/S2352-3026(20)30251-9
|
[7] |
Chan J F, Yuan S, Kok K H, et al. A familial cluster of pneumonia associated with the 2019 novel coronavirus indicating person-to-person transmission: a study of a family cluster. Lancet, 2020; 395(10223): 514-23. doi: 10.1016/S0140-6736(20)30154-9
|
[8] |
Guan W J, Ni Z Y, Hu Y, et al. Clinical characteristics of Coronavirus disease 2019 in China. N Engl J Med, 2020; 382(18): 1708-1720. doi: 10.1056/NEJMoa2002032
|
[9] |
Chan J F, Poon V K, Chan C C, et al. Low Environmental Temperature Exacerbates Severe Acute Respiratory Syndrome Coronavirus 2 Infection in Golden Syrian Hamsters. Clin Infect Dis, 2021; 75: e1101-e1111.
|
[10] |
Liu M, Li Z, Liu M, et al. Association between temperature and COVID-19 transmission in 153 countries. Environ Sci Poll Res Int, 2022; 29(11): 16017-16027. doi: 10.1007/s11356-021-16666-5
|
[11] |
Tian F, Liu X, Chao Q, et al. Ambient air pollution and low temperature associated with case fatality of COVID-19: A nationwide retrospective cohort study in China. Innovation, 2021; 2(3): 100139.
|
[12] |
Carta M G, Minerba L, Demontis R, et al. The COVID-19 incidence in Italian regions correlates with low temperature, mobility and PM10 pollution but lethality only with low temperature. J Pub Health Res, 2021; 10(4): 2303.
|
[13] |
Zhu G, Zhu Y, Wang Z, et al. The association between ambient temperature and mortality of the coronavirus disease 2019 (COVID-19) in Wuhan, China: a time-series analysis. BMC Public Health, 2021; 21(1): 117. doi: 10.1186/s12889-020-10131-7
|
[14] |
Chinazzi M, Davis J T, Ajelli M, et al. The effect of travel restrictions on the spread of the 2019 novel coronavirus (COVID-19) outbreak. Science, 2020; 368(6489): 395-400. doi: 10.1126/science.aba9757
|
[15] |
Clapp P W, Sickbert-Bennett E E, Samet J M, et al. Evaluation of Cloth Masks and Modified Procedure Masks as Personal Protective Equipment for the Public During the COVID-19 Pandemic. JAMA Intern Med, 2021; 181(4): 463-469. doi: 10.1001/jamainternmed.2020.8168
|
[16] |
Koo J R, Cook A R, Park M, et al. Interventions to mitigate early spread of SARS-CoV-2 in Singapore: a modelling study. Lancet Infect Dis, 2020; 20(6): 678-688. doi: 10.1016/S1473-3099(20)30162-6
|
[17] |
Shi Q, Hu Y, Peng B, et al. Effective control of SARS-CoV-2 transmission in Wanzhou, China. Nat Med, 2021; 27(1): 86-93. doi: 10.1038/s41591-020-01178-5
|
[18] |
Chen S, Zhang Z, Yang J, et al. Fangcang shelter hospitals: a novel concept for responding to public health emergencies. Lancet, 2020; 395(10232): 1305-1314. doi: 10.1016/S0140-6736(20)30744-3
|
[19] |
Li R, Pei S, Chen B, et al. Substantial undocumented infection facilitates the rapid dissemination of novel coronavirus (SARS-CoV-2). Science, 2020; 368(6490): 489-493. doi: 10.1126/science.abb3221
|
[20] |
Poustchi H, Darvishian M, Mohammadi Z, et al. SARS-CoV-2 antibody seroprevalence in the general population and high-risk occupational groups across 18 cities in Iran: a population-based cross-sectional study. Lancet Infect Dis, 2021; 21(4): 473-481. doi: 10.1016/S1473-3099(20)30858-6
|
[21] |
Stringhini S, Wisniak A, Piumatti G, et al. Seroprevalence of anti-SARS-CoV-2 IgG antibodies in Geneva, Switzerland (SERO-CoV-POP): a population-based study. Lancet, 2020; 396(10247): 313-319. doi: 10.1016/S0140-6736(20)31304-0
|
[22] |
Pallett S J C, Rayment M, Patel A, et al. Point-of-care serological assays for delayed SARS-CoV-2 case identification among healthcare workers in the UK: a prospective multicentre cohort study. Lancet Respir Med, 2020; 8(9): 885-894. doi: 10.1016/S2213-2600(20)30315-5
|
[23] |
Xu X, Sun J, Nie S, et al. Seroprevalence of immunoglobulin M and G antibodies against SARS-CoV-2 in China. Nat Med, 2020; 26(8): 1193-1195. doi: 10.1038/s41591-020-0949-6
|
[24] |
Li C, Luo F, Liu C, et al. Effect of a genetically engineered interferon-alpha versus traditional interferon-alpha in the treatment of moderate-to-severe COVID-19: a randomised clinical trial. Ann Med, 2021; 53(1): 391-401. doi: 10.1080/07853890.2021.1890329
|
[25] |
Bajema K L, Wiegand R E, Cuffe K, et al. Estimated SARS-CoV-2 Seroprevalence in the US as of September 2020. JAMA Intern Med, 2021; 181(4): 450-460. doi: 10.1001/jamainternmed.2020.7976
|
[26] |
Hallal P C, Hartwig F P, Horta B L, et al. SARS-CoV-2 antibody prevalence in Brazil: results from two successive nationwide serological household surveys. Lancet Glob Health, 2020; 8(11): e1390-e1398. doi: 10.1016/S2214-109X(20)30387-9
|
[27] |
Zhou P, Yang X L, Wang X G, et al. A pneumonia outbreak associated with a new coronavirus of probable bat origin. Nature, 2020; 579(7798): 270-273. doi: 10.1038/s41586-020-2012-7
|
[28] |
Wu F, Zhao S, Yu B, et al. A new coronavirus associated with human respiratory disease in China. Nature, 2020; 579(7798): 265-269. doi: 10.1038/s41586-020-2008-3
|
[29] |
McCallum M, De Marco A, Lempp FA, et al. N-terminal domain antigenic mapping reveals a site of vulnerability for SARS-CoV-2. Cell, 2021; 184(9): 2332-2347. doi: 10.1016/j.cell.2021.03.028
|
[30] |
Cerutti G, Guo Y, Zhou T, et al. Potent SARS-CoV-2 neutralizing antibodies directed against spike N-terminal domain target a single supersite. Cell host Microbe, 2021; 29: 819-833. doi: 10.1016/j.chom.2021.03.005
|
[31] |
Chi X, Yan R, Zhang J, et al. A neutralizing human antibody binds to the N-terminal domain of the Spike protein of SARS-CoV-2. Science, 2020; 369(6504): 650-655. doi: 10.1126/science.abc6952
|
[32] |
Liu L, Wang P, Nair M S, et al. Potent neutralizing antibodies against multiple epitopes on SARS-CoV-2 spike. Nature, 2020; 584(7821): 450-456. doi: 10.1038/s41586-020-2571-7
|
[33] |
Johnson B A, Xie X, Bailey A L, et al. Loss of furin cleavage site attenuates SARS-CoV-2 pathogenesis. Nature, 2021; 591(7849): 293-299. doi: 10.1038/s41586-021-03237-4
|
[34] |
Zhou Z, Huang C, Zhou Z, et al. Structural insight reveals SARS-CoV-2 ORF7a as an immunomodulating factor for human CD14(+) monocytes. iScience, 2021; 24(3): 102187. doi: 10.1016/j.isci.2021.102187
|
[35] |
Golden J W, Cline C R, Zeng X, et al. Human angiotensin-converting enzyme 2 transgenic mice infected with SARS-CoV-2 develop severe and fatal respiratory disease. JCI Insight, 2020; 5(19): e142032. doi: 10.1172/jci.insight.142032
|
[36] |
Bao L, Deng W, Huang B, et al. The pathogenicity of SARS-CoV-2 in hACE2 transgenic mice. Nature, 2020; 583(7818): 830-833. doi: 10.1038/s41586-020-2312-y
|
[37] |
Jiang R D, Liu M Q, Chen Y, et al. Pathogenesis of SARS-CoV-2 in transgenic mice expressing Human Angiotensin-Converting Enzyme 2. Cell, 2020; 182(1): 50-58. doi: 10.1016/j.cell.2020.05.027
|
[38] |
Zheng J, Wong LR, Li K, et al. COVID-19 treatments and pathogenesis including anosmia in K18-hACE2 mice. Nature, 2021; 589(7843): 603-607. doi: 10.1038/s41586-020-2943-z
|
[39] |
Dinnon K H 3rd, Leist S R, Schäfer A, et al. A mouse-adapted model of SARS-CoV-2 to test COVID-19 countermeasures. Nature, 2020; 586(7830): 560-566. doi: 10.1038/s41586-020-2708-8
|
[40] |
Rathnasinghe R, Strohmeier S, Amanat F, et al. Comparison of transgenic and adenovirus hACE2 mouse models for SARS-CoV-2 infection. Emerg Microbes Infect, 2020; 9(1): 2433-2445. doi: 10.1080/22221751.2020.1838955
|
[41] |
Sun J, Zhuang Z, Zheng J, et al. Generation of a broadly useful model for COVID-19 pathogenesis, vaccination, and treatment. Cell, 2020; 182(3): 734-743. doi: 10.1016/j.cell.2020.06.010
|
[42] |
Munster V J, Feldmann F, Williamson B N, et al. Respiratory disease in rhesus macaques inoculated with SARS-CoV-2. Nature, 2020; 585(7824): 268-272. doi: 10.1038/s41586-020-2324-7
|
[43] |
Deng W, Bao L, Gao H, et al. Ocular conjunctival inoculation of SARS-CoV-2 can cause mild COVID-19 in rhesus macaques. Nat Comm, 2020; 11(1): 4400. doi: 10.1038/s41467-020-18149-6
|
[44] |
Chandrashekar A, Liu J, Martinot A J, et al. SARS-CoV-2 infection protects against rechallenge in rhesus macaques. Science, 2020; 369(6505): 812-817. doi: 10.1126/science.abc4776
|
[45] |
Feng L, Wang Q, Shan C, et al. An adenovirus-vectored COVID-19 vaccine confers protection from SARS-COV-2 challenge in rhesus macaques. Nat Comm, 2020; 11(1): 4207. doi: 10.1038/s41467-020-18077-5
|
[46] |
Yu J, Tostanoski L H, Peter L, et al. DNA vaccine protection against SARS-CoV-2 in rhesus macaques. Science, 2020; 369(6505): 806-11. doi: 10.1126/science.abc6284
|
[47] |
Yuan S, Yin X, Meng X, et al. Clofazimine broadly inhibits coronaviruses including SARS-CoV-2. Nature, 2021; 593: 418-423. doi: 10.1038/s41586-021-03431-4
|
[48] |
Fox S E, Akmatbekov A, Harbert J, et al. Pulmonary and cardiac pathology in African American patients with COVID-19: an autopsy series from New Orleans. Lancet Respir Med, 2020; 8(7): 681-686. doi: 10.1016/S2213-2600(20)30243-5
|
[49] |
Schurink B, Roos E, Radonic T, et al. Viral presence and immunopathology in patients with lethal COVID-19: a prospective autopsy cohort study. Lancet Microbe, 2020; 1(7): e290-e299. doi: 10.1016/S2666-5247(20)30144-0
|
[50] |
Ackermann M, Verleden S E, Kuehnel M, et al. Pulmonary vascular endothelialitis, thrombosis, and angiogenesis in Covid-19. N Engl J Med, 2020; 383(2): 120-128. doi: 10.1056/NEJMoa2015432
|
[51] |
McGonagle D, O'Donnell J S, Sharif K, et al. Immune mechanisms of pulmonary intravascular coagulopathy in COVID-19 pneumonia. Lancet Rheumatol, 2020; 2(7): e437-e445. doi: 10.1016/S2665-9913(20)30121-1
|
[52] |
Hanley B, Naresh K N, Roufosse C, et al. Histopathological findings and viral tropism in UK patients with severe fatal COVID-19: a post-mortem study. Lancet Microbe, 2020; 1(6): e245-e253. doi: 10.1016/S2666-5247(20)30115-4
|
[53] |
Puelles V G, Lütgehetmann M, Lindenmeyer M T, et al. Multiorgan and Renal Tropism of SARS-CoV-2. N Engl J Med, 2020; 383(6): 590-592. doi: 10.1056/NEJMc2011400
|
[54] |
Li H, Liu L, Zhang D, et al. SARS-CoV-2 and viral sepsis: observations and hypotheses. Lancet, 2020; 395(10235): 1517-1520. doi: 10.1016/S0140-6736(20)30920-X
|
[55] |
Feng E, Balint E, Poznanski S M, et al. Aging and interferons: impacts on inflammation and viral disease outcomes. Cells, 2021; 10(3): 708. doi: 10.3390/cells10030708
|
[56] |
Ballow M, Haga C L. Why do some people develop serious COVID-19 Disease after infection, while others only exhibit mild symptoms? J Allergy Clin Immunol Pract, 2021; 9(4): 1442-1448. doi: 10.1016/j.jaip.2021.01.012
|
[57] |
Fajgenbaum D C, June C H. Cytokine storm. N Engl J Med, 2020; 383(23): 2255-2273. doi: 10.1056/NEJMra2026131
|
[58] |
Jiang L, Tang K, Levin M, et al. COVID-19 and multisystem inflammatory syndrome in children and adolescents. Lancet Infect dis, 2020; 20(11): e276-288. doi: 10.1016/S1473-3099(20)30651-4
|
[59] |
Wölfel R, Corman V M, Guggemos W, et al. Virological assessment of hospitalized patients with COVID-2019. Nature, 2020; 581(7809): 465-469. doi: 10.1038/s41586-020-2196-x
|
[60] |
He X, Lau E H Y, Wu P, et al. Temporal dynamics in viral shedding and transmissibility of COVID-19. Nat Med, 2020; 26(5): 672-675. doi: 10.1038/s41591-020-0869-5
|
[61] |
To K K, Tsang O T, Leung W S, et al. Temporal profiles of viral load in posterior oropharyngeal saliva samples and serum antibody responses during infection by SARS-CoV-2: an observational cohort study. Lancet Infect Dis, 2020; 20(5): 565-574. doi: 10.1016/S1473-3099(20)30196-1
|
[62] |
Guo W L, Jiang Q, Ye F, et al. Effect of throat washings on detection of 2019 Novel Coronavirus. Clin Infect Dis, 2020; 71(8): 1980-1981. doi: 10.1093/cid/ciaa416
|
[63] |
Shan D, Johnson J M, Fernandes S C, et al. N-protein presents early in blood, dried blood and saliva during asymptomatic and symptomatic SARS-CoV-2 infection. Nat Comm, 2021; 12(1): 1931. doi: 10.1038/s41467-021-22072-9
|
[64] |
Cevik M, Tate M, Lloyd O, et al. SARS-CoV-2, SARS-CoV, and MERS-CoV viral load dynamics, duration of viral shedding, and infectiousness: a systematic review and meta-analysis. Lancet Microbe, 2021; 2(1): e13-22. doi: 10.1016/S2666-5247(20)30172-5
|
[65] |
Wang Y, Zhang L, Sang L, et al. Kinetics of viral load and antibody response in relation to COVID-19 severity. J Clin Invest, 2020; 130(10): 5235-5244. doi: 10.1172/JCI138759
|
[66] |
Hong K, Cao W, Liu Z, et al. Prolonged presence of viral nucleic acid in clinically recovered COVID-19 patients was not associated with effective infectiousness. Emerg Microbe Infect, 2020; 9(1): 2315-2321. doi: 10.1080/22221751.2020.1827983
|
[67] |
Boum Y, Fai K N, Nicolay B, et al. Performance and operational feasibility of antigen and antibody rapid diagnostic tests for COVID-19 in symptomatic and asymptomatic patients in Cameroon: a clinical, prospective, diagnostic accuracy study. Lancet Infect Dis, 2021; 21: 1089-1096. doi: 10.1016/S1473-3099(21)00132-8
|
[68] |
Long Q X, Liu B Z, Deng H J, et al. Antibody responses to SARS-CoV-2 in patients with COVID-19. Natmed, 2020; 26(6): 845-848.
|
[69] |
Yu H Q, Sun B Q, Fang Z F, et al. Distinct features of SARS-CoV-2-specific IgA response in COVID-19 patients. Eur Respir J, 2020; 56(2): 2001526. doi: 10.1183/13993003.01526-2020
|
[70] |
Sterlin D, Mathian A, Miyara M, et al. IgA dominates the early neutralizing antibody response to SARS-CoV-2. Sci Transl Med, 2021; 13(577): eabd2223. doi: 10.1126/scitranslmed.abd2223
|
[71] |
Chen M, Qin R, Jiang M, et al. Clinical applications of detecting IgG, IgM or IgA antibody for the diagnosis of COVID-19: A meta-analysis and systematic review. Int J Infect Dis, 2021; 104: 415-422. doi: 10.1016/j.ijid.2021.01.016
|
[72] |
Niu X, Li S, Li P, et al. Longitudinal analysis of T and B cell receptor repertoire transcripts reveal dynamic immune response in COVID-19 patients. Front Immunol, 2020; 11: 582010. doi: 10.3389/fimmu.2020.582010
|
[73] |
Wilk A J, Rustagi A, Zhao N Q, et al. A single-cell atlas of the peripheral immune response in patients with severe COVID-19. Nat Med, 2020; 26(7): 1070. doi: 10.1038/s41591-020-0944-y
|
[74] |
Bost P, Giladi A, Liu Y, et al. Host-Viral infection maps reveal signatures of severe COVID-19 Patients. Cell, 2020; 181(7): 1475-1488. doi: 10.1016/j.cell.2020.05.006
|
[75] |
Liao M, Liu Y, Yuan J, et al. Single-cell landscape of bronchoalveolar immune cells in patients with COVID-19. Nat Med, 2020; 26(6): 842-844. doi: 10.1038/s41591-020-0901-9
|
[76] |
Dejnirattisai W, Zhou D, Supasa P, et al. Antibody evasion by the P. 1 strain of SARS-CoV-2. Cell, 2021; 184: 2939-2954. doi: 10.1016/j.cell.2021.03.055
|
[77] |
Emary K R W, Golubchik T, Aley P K, et al. Efficacy of ChAdOx1 nCoV-19 (AZD1222) vaccine against SARS-CoV-2 variant of concern 202012/01 (B.1.1.7): an exploratory analysis of a randomised controlled trial. Lancet, 2021; 397(10282): 1351-1362. doi: 10.1016/S0140-6736(21)00628-0
|
[78] |
Challen R, Brooks-Pollock E, Read J M, et al. Risk of mortality in patients infected with SARS-CoV-2 variant of concern 202012/1: matched cohort study. BMJ, 2021; 372: n579.
|
[79] |
Frampton D, Rampling T, Cross A, et al. Genomic characteristics and clinical effect of the emergent SARS-CoV-2 B.1.1.7 lineage in London, UK: a whole-genome sequencing and hospital-based cohort study. Lancet Infect Dis, 2021; 21: 1246-1256. doi: 10.1016/S1473-3099(21)00170-5
|
[80] |
Keehner J, Horton L E, Pfeffer M A, et al. SARS-CoV-2 infection after vaccination in health care workers in California. N Engl J Med, 2021; 384(18): 1774-1775. doi: 10.1056/NEJMc2101927
|
[81] |
Li Q, Nie J, Wu J, et al. SARS-CoV-2 501Y. V2 variants lack higher infectivity but do have immune escape. Cell, 2021; 184(9): 2362-2371. doi: 10.1016/j.cell.2021.02.042
|
[82] |
Zhou D, Dejnirattisai W, Supasa P, et al. Evidence of escape of SARS-CoV-2 variant B.1.351 from natural and vaccine-induced sera. Cell, 2021; 184(9): 2348-2461. doi: 10.1016/j.cell.2021.02.037
|
[83] |
Hoffmann M, Arora P, Groß R, et al. SARS-CoV-2 variants B.1.351 and P.1 escape from neutralizing antibodies. Cell, 2021; 184(9): 2384-2393. doi: 10.1016/j.cell.2021.03.036
|
[84] |
Wolter N, Jassat W, Walaza S, et al. Early assessment of the clinical severity of the SARS-CoV-2 omicron variant in South Africa: a data linkage study. Lancet, 2022; 399(10323): 437-446. doi: 10.1016/S0140-6736(22)00017-4
|
[85] |
Abu-Raddad L J, Chemaitelly H, Butt A A. Effectiveness of the BNT162b2 Covid-19 Vaccine against the B.1.1.7 and B.1.351 Variants. BMJ Med, 2022; 12(3): 386.
|
[86] |
Shinde V, Bhikha S, Hoosain Z, et al. Efficacy of NVX-CoV2373 Covid-19 Vaccine against the B.1.351 Variant. N Engl J Med, 2021; 384: 1899-1909. doi: 10.1056/NEJMoa2103055
|
[87] |
Shen X, Tang H, Pajon R, et al. Neutralization of SARS-CoV-2 variants B.1.429 and B.1.351. N Engl J Med, 2021; 384: 2352-2354. doi: 10.1056/NEJMc2103740
|
[88] |
Supasa P, Zhou D, Dejnirattisai W, et al. Reduced neutralization of SARS-CoV-2 B.1.1.7 variant by convalescent and vaccine sera. Cell, 2021; 184(8): 2201-2211. doi: 10.1016/j.cell.2021.02.033
|
[89] |
Madhi S A, Baillie V, Cutland C L, et al. Efficacy of the ChAdOx1 nCoV-19 Covid-19 vaccine against the B.1.351 variant. N Engl J Med, 2021; 384: 1885-1898. doi: 10.1056/NEJMoa2102214
|
[90] |
Moyo-Gwete T, Madzivhandila M, Makhado Z, et al. Cross-Reactive neutralizing antibody responses elicited by SARS-CoV-2 501Y. V2 (B.1.351). N Engl J Med, 2021; 384: 2161-2163. doi: 10.1056/NEJMc2104192
|
[91] |
Wang G L, Wang Z Y, Duan L J, et al. Susceptibility of circulating SARS-CoV-2 variants to Neutralization. N Engl J Med, 2021; 384: 2354-2356. doi: 10.1056/NEJMc2103022
|
[92] |
Lustig Y, Nemet I, Kliker L, et al. Neutralizing response against variants after SARS-CoV-2 infection and one dose of BNT162b2. N Engl J Med, 2021; 384: 2453-2454. doi: 10.1056/NEJMc2104036
|
[93] |
Andrews N, Stowe J, Kirsebom F, et al. Covid-19 vaccine effectiveness against the Omicron (B.1.1.529) variant. N Engl J Med, 2022; 386: 1532-1546. doi: 10.1056/NEJMoa2119451
|
[94] |
Pajon R, Doria-Rose N A, Shen X, et al. SARS-CoV-2 Omicron variant neutralization after mRNA-1273 booster vaccination. N Engl J Med, 2022; 386(11): 1088-1091. doi: 10.1056/NEJMc2119912
|
[95] |
Regev-Yochay G, Gonen T, Gilboa M, , et al. Efficacy of a fourth dose of Covid-19 mRNA Vaccine against Omicron. N Engl J Med, 2022; 386: 1377-1380. doi: 10.1056/NEJMc2202542
|
[96] |
Chia W N, Zhu F, Ong S W X, et al. Dynamics of SARS-CoV-2 neutralising antibody responses and duration of immunity: a longitudinal study. Lancet Microbe, 2021; 2: e240-249. doi: 10.1016/S2666-5247(21)00025-2
|
[97] |
Hansen C H, Michlmayr D, Gubbels S M, et al. Assessment of protection against reinfection with SARS-CoV-2 among 4 million PCR-tested individuals in Denmark in 2020: a population-level observational study. Lancet, 2021; 397(10280): 1204-1212. doi: 10.1016/S0140-6736(21)00575-4
|
[98] |
Hall V J, Foulkes S, Charlett A, et al. SARS-CoV-2 infection rates of antibody-positive compared with antibody-negative health-care workers in England: a large, multicentre, prospective cohort study (SIREN). Lancet, 2021; 397(10283): 1459-1469. doi: 10.1016/S0140-6736(21)00675-9
|
[99] |
Letizia A G, Ge Y, Vangeti S, et al. SARS-CoV-2 seropositivity and subsequent infection risk in healthy young adults: a prospective cohort study. Lancet Respir Med, 2021; 9: 712-720. doi: 10.1016/S2213-2600(21)00158-2
|
[100] |
Chen N, Zhou M, Dong X, et al. Epidemiological and clinical characteristics of 99 cases of 2019 novel coronavirus pneumonia in Wuhan, China: a descriptive study. Lancet, 2020; 395(10223): 507-513. doi: 10.1016/S0140-6736(20)30211-7
|
[101] |
Wang D, Hu B, Hu C, et al. Clinical characteristics of 138 hospitalized patients with 2019 Novel Coronavirus-Infected Pneumonia in Wuhan, China. JAMA, 2020; 323(11): 1061-1069. doi: 10.1001/jama.2020.1585
|
[102] |
Feldstein L R, Rose E B, Horwitz S M, et al. Multisystem inflammatory syndrome in U.S. children and adolescents. N Engl J Med, 2020; 383(4): 334-346. doi: 10.1056/NEJMoa2021680
|
[103] |
Shi H, Han X, Jiang N, et al. Radiological findings from 81 patients with COVID-19 pneumonia in Wuhan, China: a descriptive study. Lancet Infect Dis, 2020; 20(4): 425-434. doi: 10.1016/S1473-3099(20)30086-4
|
[104] |
Zhou F, Yu T, Du R, et al. Clinical course and risk factors for mortality of adult inpatients with COVID-19 in Wuhan, China: a retrospective cohort study. Lancet, 2020; 395(10229): 1054-1062. doi: 10.1016/S0140-6736(20)30566-3
|
[105] |
Yanes-Lane M, Winters N, Fregonese F, et al. Proportion of asymptomatic infection among COVID-19 positive persons and their transmission potential: a systematic review and meta-analysis. PloS One, 2020; 15(11): e0241536. doi: 10.1371/journal.pone.0241536
|
[106] |
Long Q X, Tang X J, Shi Q L, et al. Clinical and immunological assessment of asymptomatic SARS-CoV-2 infections. Nat Med, 2020; 26(8): 1200-1204. doi: 10.1038/s41591-020-0965-6
|
[107] |
Yang M, Li L, Huang T, et al. SARS-CoV-2 detected on environmental fomites for both asymptomatic and symptomatic patients with COVID-19. Am J Respir Crit Care Med, 2021; 203(3): 374-378. doi: 10.1164/rccm.202006-2136LE
|
[108] |
Melis M, Littera R. Undetected infectives in the Covid-19 pandemic. Int J Infect Dis, 2021; 104: 262-268. doi: 10.1016/j.ijid.2021.01.010
|
[109] |
Davies N G, Klepac P, Liu Y, et al. Age-dependent effects in the transmission and control of COVID-19 epidemics. Nat Med, 2020; 26(8): 1205-1211. doi: 10.1038/s41591-020-0962-9
|
[110] |
Ahrenfeldt L J, Otavova M, Christensen K, et al. Sex and age differences in COVID-19 mortality in Europe Res Sq, 2020; rs. 3. rs-61444.
|
[111] |
Torres Acosta M A, Singer B D. Pathogenesis of COVID-19-induced ARDS: implications for an ageing population. Eur Respir J, 2020; 56(3): 2002049. doi: 10.1183/13993003.02049-2020
|
[112] |
Koff W C, Williams M A. Covid-19 and immunity in aging populations - a new research agenda. N Engl J Med, 2020; 383(9): 804-805. doi: 10.1056/NEJMp2006761
|
[113] |
Wang X Q, Song G, Yang Z, et al. Association between ageing population, median age, life expectancy and mortality in coronavirus disease (COVID-19). Aging, 2020; 12(24): 24570-24578. doi: 10.18632/aging.104193
|
[114] |
Imam Z, Odish F, Gill I, et al. Older age and comorbidity are independent mortality predictors in a large cohort of 1305 COVID-19 patients in Michigan, United States. J Intern Med, 2020; 288(4): 469-476. doi: 10.1111/joim.13119
|
[115] |
Guan W J, Laing W H, Zhao Y, et al. Comorbidity and its impact on 1590 patients with COVID-19 in China: a nationwide analysis. Eur Respir J, 2020; 55(5): 2000547. doi: 10.1183/13993003.00547-2020
|
[116] |
Liang W, Guan W, Chen R, et al. Cancer patients in SARS-CoV-2 fection: a nationwide analysis in China. Lancet Oncol, 2020; 21(3): 335-337. doi: 10.1016/S1470-2045(20)30096-6
|
[117] |
Guan W J, Liang W H, Shi Y, et al. Chronic respiratory diseases and the outcomes of COVID-19: a nationwide retrospective cohort study of 39, 420 Cases. J Allergy Clin Immunol Pract, 2021; 9: 2645-2655. doi: 10.1016/j.jaip.2021.02.041
|
[118] |
Bloom C I, Drake T M, Docherty A B, et al. Risk of adverse outcomes in patients with underlying respiratory conditions admitted to hospital with COVID-19: a national, multicentre prospective cohort study using the ISARIC WHO Clinical Characterisation Protocol UK. Lancet Respir Med, 2021; 9: 699-711. doi: 10.1016/S2213-2600(21)00013-8
|
[119] |
Calmes D, Graff S, Maes N, et al. Asthma and COPD are not risk factors for ICU stay and death in case of SARS-CoV2 Infection. J Allergy Clin Immunol Pract, 2021; 9(1): 160-169. doi: 10.1016/j.jaip.2020.09.044
|
[120] |
Skevaki C, Karsonova A, Karaulov A, et al. Asthma-associated risk for COVID-19 development. J Allergy Clin Immunol, 2020; 146(6): 1295-1301. doi: 10.1016/j.jaci.2020.09.017
|
[121] |
Song J, Zeng M, Wang H, et al. Distinct effects of asthma and COPD comorbidity on disease expression and outcome in patients with COVID-19. Allergy, 2021; 76(2): 483-496. doi: 10.1111/all.14517
|
[122] |
World Health Organization. Clinical management of COVID-19: interim guidance, 27 May 2020.
|
[123] |
Wei P F. Diagnosis and Treatment Protocol for Novel Coronavirus Pneumonia (Trial Version 7). Chin Med J (Engl), 2020; 133(9): 1087-1095. doi: 10.1097/CM9.0000000000000819
|
[124] |
International Severe Acute Respiratory and emerging Infection Consortium.
|
[125] |
Zhang X, Tan Y, Ling Y, et al. Viral and host factors related to the clinical outcome of COVID-19. Nature, 2020; 583(7816): 437-40. doi: 10.1038/s41586-020-2355-0
|
[126] |
Chen R, Sang L, Jiang M, et al. Longitudinal hematologic and immunologic variations associated with the progression of COVID-19 patients in China. J Allergy Clin Immunol, 2020; 146(1): 89-100. doi: 10.1016/j.jaci.2020.05.003
|
[127] |
Shen B, Yi X, Sun Y. et al. Proteomic and metabolomic characterization of COVID-19 patient sera. Cell, 2020; 182(1): 59-72. doi: 10.1016/j.cell.2020.05.032
|
[128] |
Ren L, Wang Y, Zhong J, et al. Dynamics of the upper respiratory tract microbiota and its association with mortality in COVID-19. Am J Respir Crit Care Med, 2021; 204(12): 1379-1390. doi: 10.1164/rccm.202103-0814OC
|
[129] |
Liang W, Liang H, Ou L, et al. Development and validation of a clinical risk score to predict the occurrence of critical illness in hospitalized patients with COVID-19. JAMA Intern Med, 2020; 180(8): 1081-9. doi: 10.1001/jamainternmed.2020.2033
|
[130] |
Alemany A, Bar C B, Ouchi D, et al. Analytical and clinical performance of the panbio COVID-19 antigen-detecting rapid diagnostic test. J Infect, 2021; 82(5): 186-230.
|
[131] |
Kitagawa Y, Orihara Y, Kawamura R, et al. Evaluation of rapid diagnosis of novel coronavirus disease (COVID-19) using loop-mediated isothermal amplification. J Clin Virol, 2020; 129: 104446. doi: 10.1016/j.jcv.2020.104446
|
[132] |
Xing W, Liu Y, Wang H, et al. A high-throughput, multi-index isothermal amplification platform for rapid detection of 19 types of common respiratory viruses including SARS-CoV-2. Engineering, 2020; 6(10): 1130-1140. doi: 10.1016/j.eng.2020.07.015
|
[133] |
Xing W, Wang J, Zhao C, et al. A highly automated mobile laboratory for on-site molecular diagnostics in the COVID-19 pandemic. Clin Chem, 2021; 67(4): 672-683. doi: 10.1093/clinchem/hvab027
|
[134] |
Joung J, Ladha A, Saito M, et al. Detection of SARS-CoV-2 with sherlock one-pot testing. N Engl J Med, 2020; 383(15): 1492-1494. doi: 10.1056/NEJMc2026172
|
[135] |
Brendish N J, Poole S, Naidu V V, et al. Clinical impact of molecular point-of-care testing for suspected COVID-19 in hospital (COV-19POC): a prospective, interventional, non-randomised, controlled study. Lancet Respir Med, 2020; 8(12): 1192-1200. doi: 10.1016/S2213-2600(20)30454-9
|
[136] |
Gibani M M, Toumazou C, Sohbati M, et al. Assessing a novel, lab-free, point-of-care test for SARS-CoV-2 (CovidNudge): a diagnostic accuracy study. Lancet Microbe, 2020; 1(7): e300-307. doi: 10.1016/S2666-5247(20)30121-X
|
[137] |
Zhang K, Liu X, Shen J, et al. Clinically applicable AI system for accurate diagnosis, quantitative measurements, and prognosis of COVID-19 pneumonia using computed tomography. Cell, 2020; 182(5): 1360. doi: 10.1016/j.cell.2020.08.029
|
[138] |
Mei X, Lee H C, Diao K Y, et al. Artificial intelligence-enabled rapid diagnosis of patients with COVID-19. Nat Med, 2020; 26(8): 1224-1228. doi: 10.1038/s41591-020-0931-3
|
[139] |
Jiao Z, Choi J W, Halsey K, et al. Prognostication of patients with COVID-19 using artificial intelligence based on chest x-rays and clinical data: a retrospective study. Lancet Digit Health, 2021; 3(5): e286-294. doi: 10.1016/S2589-7500(21)00039-X
|
[140] |
Wang G, Liu X, Shen J, et al. A deep-learning pipeline for the diagnosis and discrimination of viral, non-viral and COVID-19 pneumonia from chest X-ray images. Nat Biomed Eng, 2021; 5: 943. doi: 10.1038/s41551-021-00787-w
|
[141] |
Menni C, Valdes A M, Freidin M B, et al. Real-time tracking of self-reported symptoms to predict potential COVID-19. Nat Med, 2020; 26(7): 1037-1040. doi: 10.1038/s41591-020-0916-2
|
[142] |
Mendels D A, Dortet L, Emeraud C, et al. Using artificial intelligence to improve COVID-19 rapid diagnostic test result interpretation. Proc Natl Aca Sci, 2021; 118(12): : e2019893118. doi: 10.1073/pnas.2019893118
|
[143] |
Cao B, Wang Y, Wen D, et al. A trial of lopinavir-ritonavir in adults hospitalized with severe Covid-19. N Engl J Med, 2020; 382(19): 1787-1799. doi: 10.1056/NEJMoa2001282
|
[144] |
Recovery Collaborative Group. Lopinavir-ritonavir in patients admitted to hospital with COVID-19 (Recovery): a randomised, controlled, open-label, platform trial. Lancet, 2020; 396(10259): 1345-1352. doi: 10.1016/S0140-6736(20)32013-4
|
[145] |
Panerai S, Raggi A, Tasca D, et al. Telephone-based reality orientation therapy for patients with dementia: a pilot study during the COVID-19 outbreak. Am J Occup Ther, 2021; 75(2): 1-9.
|
[146] |
Hung I F, Lung K C, Tso E Y, et al. Triple combination of interferon beta-1b, lopinavir-ritonavir, and ribavirin in the treatment of patients admitted to hospital with COVID-19: an open-label, randomised, phase 2 trial. Lancet, 2020; 395(10238): 1695-1704. doi: 10.1016/S0140-6736(20)31042-4
|
[147] |
Hammond J, Leister-Tebbe H, Gardner A, et al. Oral nirmatrelvir for high-risk, nonhospitalized adults with Covid-19. N Engl J Med, 2022; 386: 1397-1408. doi: 10.1056/NEJMoa2118542
|
[148] |
Goldman J D, Lye D C B, Hui D S, et al. Remdesivir for 5 or 10 Days in patients with severe Covid-19. N Engl J Med, 2020; 383(19): 1827-1837. doi: 10.1056/NEJMoa2015301
|
[149] |
Wang Y, Zhang D, Du G, et al. Remdesivir in adults with severe COVID-19: a randomised, double-blind, placebo-controlled, multicentre trial. Lancet, 2020; 395(10236): 1569-1578. doi: 10.1016/S0140-6736(20)31022-9
|
[150] |
Beigel J H, Tomashek K M, Dodd L E, et al. Remdesivir for the treatment of Covid-19-final report. N Engl J Med, 2020; 383(19): 1813-1826. doi: 10.1056/NEJMoa2007764
|
[151] |
Spinner C D, Gottlieb R L, Criner G J, et al. Effect of remdesivir vs standard care on clinical status at 11 days in patients with moderate COVID-19: a randomized clinical trial. JAMA, 2020; 324(11): 1048-1057. doi: 10.1001/jama.2020.16349
|
[152] |
Yan D, Liu X Y, Zhu Y N, et al. Factors associated with prolonged viral shedding and impact of lopinavir/ritonavir treatment in hospitalised non-critically ill patients with SARS-CoV-2 infection. Eur Respir J, 2020; 56(1): 2000799. doi: 10.1183/13993003.00799-2020
|
[153] |
Gottlieb R L, Vaca C E, Paredes R, et al. Early Remdesivir to prevent progression to severe Covid-19 in outpatients. N Engl J Med, 2022; 386(4): 305-315. doi: 10.1056/NEJMoa2116846
|
[154] |
Jayk Bernal A, Gomes da Silva M M, Musungaie D B, et al. Molnupiravir for oral treatment of Covid-19 in nonhospitalized patients. N Engl J Med, 2022; 386(6): 509-520. doi: 10.1056/NEJMoa2116044
|
[155] |
Reis G, Silva E, Silva D C M, et al. Effect of early treatment with ivermectin among patients with Covid-19. N Engl J Med, 2022; 386: 1721-1731. doi: 10.1056/NEJMoa2115869
|
[156] |
Cavalcanti A B, Zampieri FG, Rosa R G, et al. Hydroxychloroquine with or without Azithromycin in Mild-to-Moderate Covid-19. N Engl J Med, 2020; 383(21): e119. doi: 10.1056/NEJMx200021
|
[157] |
Self W H, Semler M W, Leither L M, et al. Effect of hydroxychloroquine on clinical status at 14 days in hospitalized patients with COVID-19: a randomized clinical trial. JAMA, 2020; 324(21): 2165-2176. doi: 10.1001/jama.2020.22240
|
[158] |
Tang W, Cao Z, Han M, et al. Hydroxychloroquine in patients with mainly mild to moderate coronavirus disease 2019: open label, randomised controlled trial. BMJ, 2020; 369: m1849.
|
[159] |
Horby P, Mafham M, Linsell L, et al. Effect of hydroxychloroquine in hospitalized patients with Covid-19. N Engl J Med, 2020; 383(21): 2030-2040. doi: 10.1056/NEJMoa2022926
|
[160] |
Boulware D R, Pullen M F, Bangdiwala A S, et al. A randomized trial of hydroxychloroquine as postexposure prophylaxis for Covid-19. N Engl J Med, 2020; 383(6): 517-525. doi: 10.1056/NEJMoa2016638
|
[161] |
Mitjà O, Corbacho-Monné M, Ubals M, et al. A cluster-randomized trial of hydroxychloroquine for prevention of Covid-19. N Engl J Med, 2021; 384(5): 417-427. doi: 10.1056/NEJMoa2021801
|
[162] |
Zhao H, Zhang C, Zhu Q, et al. Favipiravir in the treatment of patients with SARS-CoV-2 RNA recurrent positive after discharge: a multicenter, open-label, randomized trial. Int Immunopharmacol, 2021; 97: 107702. doi: 10.1016/j.intimp.2021.107702
|
[163] |
Solaymani-Dodaran M, Ghanei M, Bagheri M, et al. Safety and efficacy of Favipiravir in moderate to severe SARS-CoV-2 pneumonia. Int Immunopharmacol, 2021; 95: 107522. doi: 10.1016/j.intimp.2021.107522
|
[164] |
López-Medina E, López P, Hurtado I C, et al. Effect of ivermectin on time to resolution of symptoms among adults with mild COVID-19: a randomized clinical trial. JAMA, 2021; 325(14): 1426-1435. doi: 10.1001/jama.2021.3071
|
[165] |
Monk P D, Marsden R J, Tear V J, et al. Safety and efficacy of inhaled nebulised interferon beta-1a (SNG001) for treatment of SARS-CoV-2 infection: a randomised, double-blind, placebo-controlled, phase 2 trial. Lancet Respir Med, 2021; 9(2): 196-206. doi: 10.1016/S2213-2600(20)30511-7
|
[166] |
Tomazini B M, Maia I S, Cavalcanti A B, et al. Effect of dexamethasone on days alive and ventilator-free in patients with moderate or severe acute respiratory distress syndrome and COVID-19: the CoDEX randomized clinical trial. JAMA, 2020; 324(13): 1307-1316. doi: 10.1001/jama.2020.17021
|
[167] |
Horby P, Lim W S, Emberson J R, et al. Dexamethasone in hospitalized patients with Covid-19. N Engl J Med, 2021; 384(8): 693-704. doi: 10.1056/NEJMoa2021436
|
[168] |
Angus D C, Derde L, Al-Beidh F, et al. Effect of hydrocortisone on mortality and organ support in patients with severe COVID-19: the REMAP-CAP COVID-19 corticosteroid domain randomized clinical trial. JAMA, 2020; 324(13): 1317-1329. doi: 10.1001/jama.2020.17022
|
[169] |
Dequin P F, Heming N, Meziani F, et al. Effect of hydrocortisone on 21-day mortality or respiratory support among Critically Ill patients with COVID-19: a randomized clinical trial. JAMA, 2020; 324(13): 1298-1306. doi: 10.1001/jama.2020.16761
|
[170] |
Wu C, Chen X, Cai Y, et al. Risk factors associated with acute respiratory distress syndrome and death in patients with Coronavirus disease 2019 pneumonia in Wuhan, China. JAMA Intern Med, 2020; 180(7): 934-943. doi: 10.1001/jamainternmed.2020.0994
|
[171] |
Kalil A C, Patterson T F, Mehta A K, et al. Baricitinib plus remdesivir for hospitalized adults with Covid-19. N Engl J Med, 2021; 384(9): 795-807. doi: 10.1056/NEJMoa2031994
|
[172] |
Lenze E J, Mattar C, Zorumski C F, et al. Fluvoxamine vs Placebo and clinical deterioration in outpatients with symptomatic COVID-19: a randomized clinical trial. JAMA, 2020; 324(22): 2292-2300. doi: 10.1001/jama.2020.22760
|
[173] |
Ramakrishnan S, Nicolau D V, Jr Langford B, et al. Inhaled budesonide in the treatment of early COVID-19 (STOIC): a phase 2, open-label, randomised controlled trial. Lancet Respir Med, 2021; 9(7): 763-772. doi: 10.1016/S2213-2600(21)00160-0
|
[174] |
Principle Trial Collaborative Group. Azithromycin for community treatment of suspected COVID-19 in people at increased risk of an adverse clinical course in the UK (Principle): a randomised, controlled, open-label, adaptive platform trial. Lancet, 2021; 397(10279): 1063-1074. doi: 10.1016/S0140-6736(21)00461-X
|
[175] |
Bhattacharya B, Kumar R, Meena V P, et al. SARS-CoV-2 RT-PCR profile in 298 Indian COVID-19 patients: a retrospective observational study. Path Dis, 2021; 79(1): 64.
|
[176] |
Furtado R H M, Berwanger O, Fonseca H A, et al. Azithromycin in addition to standard of care versus standard of care alone in the treatment of patients admitted to the hospital with severe COVID-19 in Brazil (COALITION Ⅱ): a randomised clinical trial. Lancet, 2020; 396(10256): 959-967. doi: 10.1016/S0140-6736(20)31862-6
|
[177] |
Cao Y, Wei J, Zou L, et al. Ruxolitinib in treatment of severe coronavirus disease 2019 (COVID-19): a multicenter, single-blind, randomized controlled trial. J Allergy Clin Immunol, 2020; 146(1): 137-146. doi: 10.1016/j.jaci.2020.05.019
|
[178] |
Murai I H, Fernandes A L, Sales L P, et al. Effect of a single high dose of Vitamin D3 on hospital length of stay in patients with moderate to severe COVID-19: a randomized clinical trial. JAMA, 2021; 325(11): 1053-1060. doi: 10.1001/jama.2020.26848
|
[179] |
Recovery Collaborative Group. Colchicine in patients admitted to hospital with COVID-19 (Recovery): a randomised, controlled, open-label, platform trial. Lancet Respir Med, 2021; 9(12): 1419-1426. doi: 10.1016/S2213-2600(21)00435-5
|
[180] |
Gordon A C, Mouncey P R, Al-Beidh F, et al. Interleukin-6 receptor antagonists in Critically Ill patients with Covid-19. N Engl J Med, 2021; 384(16): 1491-1502. doi: 10.1056/NEJMoa2100433
|
[181] |
Recovery Collaborative Group. Tocilizumab in patients admitted to hospital with COVID-19 (Recovery): a randomised, controlled, open-label, platform trial. Lancet, 2021; 397(10285): 1637-1645. doi: 10.1016/S0140-6736(21)00676-0
|
[182] |
Rosas I O, Bräu N, Waters M, et al. Tocilizumab in hospitalized patients with severe Covid-19 pneumonia. N Engl J Med, 2021; 384(16): 1503-1516. doi: 10.1056/NEJMoa2028700
|
[183] |
Hermine O, Mariette X, Tharaux P L, et al. Effect of tocilizumab vs usual care in adults hospitalized with COVID-19 and moderate or severe pneumonia: a randomized clinical trial. JAMA Intern Med, 2021; 181(1): 32-40. doi: 10.1001/jamainternmed.2020.6820
|
[184] |
Veiga V C, Prats J, Farias D L C, et al. Effect of tocilizumab on clinical outcomes at 15 days in patients with severe or critical coronavirus disease 2019: randomised controlled trial. BMJ, 2021; 372: n84.
|
[185] |
Soin A S, Kumar K, Choudhary N S, et al. Tocilizumab plus standard care versus standard care in patients in India with moderate to severe COVID-19-associated cytokine release syndrome (COVINTOC): an open-label, multicentre, randomised, controlled, phase 3 trial. Lancet Respir Med, 2021; 9(5): 511-521. doi: 10.1016/S2213-2600(21)00081-3
|
[186] |
Stone J H, Frigault M J, Serling-Boyd N J, et al. Efficacy of tocilizumab in patients hospitalized with Covid-19. N Engl J Med, 2020; 383(24): 2333-2344. doi: 10.1056/NEJMoa2028836
|
[187] |
Salvarani C, Dolci G, Massari M, et al. Effect of tocilizumab vs standard care on clinical worsening in patients hospitalized with COVID-19 pneumonia: a randomized clinical trial. JAMA Intern Med, 2021; 181(1): 24-31. doi: 10.1001/jamainternmed.2020.6615
|
[188] |
Lescure F X, Honda H, Fowler R A, et al. Sarilumab in patients admitted to hospital with severe or critical COVID-19: a randomised, double-blind, placebo-controlled, phase 3 trial. Lancet Respir Med, 2021; 9(5): 522-532. doi: 10.1016/S2213-2600(21)00099-0
|
[189] |
Cremer P C, Abbate A, Hudock K, et al. Mavrilimumab in patients with severe COVID-19 pneumonia and systemic hyperinflammation (MASH-COVID): an investigator initiated, multicentre, double-blind, randomised, placebo-controlled trial. Lancet Rheumatol, 2021; 3: e410-e418. doi: 10.1016/S2665-9913(21)00070-9
|
[190] |
Cheng L L, Guan W J, Duan C Y, et al. Effect of recombinant human granulocyte colony-stimulating factor for patients with Coronavirus Disease 2019 (COVID-19) and lymphopenia: a randomized clinical trial. JAMA Intern Med, 2021; 181(1): 71-78. doi: 10.1001/jamainternmed.2020.5503
|
[191] |
Okoh A K, Bishburg E, Grinberg S, et al. Tocilizumab use in COVID-19-associated pneumonia. J Med Virol, 2021; 93(2): 1023-1028. doi: 10.1002/jmv.26471ternmed.2020.5503
|
[192] |
Vlaar A P J, de Bruin S, Busch M, et al. Anti-C5a antibody IFX-1 (vilobelimab) treatment versus best supportive care for patients with severe COVID-19 (PANAMO): an exploratory, open-label, phase 2 randomised controlled trial. Lancet Rheumatol, 2020; 2(12): e764-773. doi: 10.1016/S2665-9913(20)30341-6
|
[193] |
Cheng Y, Wong R, Soo Y O, et al. Use of convalescent plasma therapy in SARS patients in Hong Kong. Eur J Clin Microbiol Infect Dis, 2005; 24(1): 44-46. doi: 10.1007/s10096-004-1271-9
|
[194] |
Weinreich D M, Sivapalasingam S, Norton T, et al. REGN-COV2, a neutralizing antibody cocktail, in outpatients with Covid-19. N Engl J Med, 2021; 384(3): 238-251. doi: 10.1056/NEJMoa2035002
|
[195] |
Wang J, Zheng X, Chen J. Clinical progression and outcomes of 260 patients with severe COVID-19: an observational study. Sci Rep, 2021; 11(1): 3166. doi: 10.1038/s41598-021-82943-5
|
[196] |
Gottlieb R L, Nirula A, Chen P, et al. Effect of bamlanivimab as monotherapy or in combination with etesevimab on viral load in patients with mild to moderate COVID-19: a randomized clinical trial. JAMA, 2021; 325(7): 632-644. doi: 10.1001/jama.2021.0202
|
[197] |
Andreano E, Nicastri E, Paciello I, et al. Extremely potent human monoclonal antibodies from COVID-19 convalescent patients. Cell, 2021; 184(7): 1821-1835. doi: 10.1016/j.cell.2021.02.035
|
[198] |
Libster R, Pérez Marc G, Wappner D, et al. Early high-titer plasma therapy to prevent severe Covid-19 in older adults. N Engl J Med, 2021; 384(7): 610-618. doi: 10.1056/NEJMoa2033700
|
[199] |
Agarwal A, Mukherjee A, Kumar G, et al. Convalescent plasma in the management of moderate covid-19 in adults in India: open label phase Ⅱ multicentre randomised controlled trial (PLACID Trial). BMJ, 2020; 371: m3939.
|
[200] |
Simonovich V A, Burgos Pratx L D, Scibona P, et al. A randomized trial of convalescent plasma in Covid-19 severe pneumonia. N Engl J Med, 2021; 384(7): 619-629. doi: 10.1056/NEJMoa2031304
|
[201] |
Lundgren J D, Grund B, Barkauskas C E, et al. A neutralizing monoclonal antibody for hospitalized patients with Covid-19. N Engl J Med, 2021; 384(10): 905-914. doi: 10.1056/NEJMoa2033130
|
[202] |
Li L, Zhang W, Hu Y, et al. Effect of convalescent plasma therapy on time to clinical improvement in patients with severe and life-threatening COVID-19: a randomized clinical trial. JAMA, 2020; 324(5): 460-470. doi: 10.1001/jama.2020.10044
|
[203] |
Janiaud P, Axfors C, Schmitt A M, et al. Association of convalescent plasma treatment with clinical outcomes in patients with COVID-19: a systematic review and meta-analysis. JAMA, 2021; 325(12): 1185-1195. doi: 10.1001/jama.2021.2747
|
[204] |
Sullivan D J, Gebo K A, Shoham S, et al. Early outpatient treatment for Covid-19 with convalescent plasma. N Engl J Med, 2022; 386: 1700-1711. doi: 10.1056/NEJMoa2119657
|
[205] |
Mancia G, Rea F, Ludergnani M, et al. Renin-Angiotensin-Aldosterone system blockers and the risk of Covid-19. N Engl J Med, 2020; 382(25): 2431-2440. doi: 10.1056/NEJMoa2006923
|
[206] |
Reynolds H R, Adhikari S, Pulgarin C, et al. Renin-Angiotensin-Aldosterone system inhibitors and risk of Covid-19. N Engl J Med, 2020; 382(25): 2441-2448. doi: 10.1056/NEJMoa2008975
|
[207] |
Morales D R, Conover M M, You S C, et al. Renin-angiotensin system blockers and susceptibility to COVID-19: an international, open science, cohort analysis. Lancet Digit health, 2021; 3(2): e98-114. doi: 10.1016/S2589-7500(20)30289-2
|
[208] |
=Mehta N, Kalra A, Nowacki A S, et al. Association of use of angiotensin-converting enzyme inhibitors and Angiotensin Ⅱ receptor blockers with testing positive for Coronavirus Disease 2019 (COVID-19). JAMA Cardiol, 2020; 5(9): 1020-1026. doi: 10.1001/jamacardio.2020.1855
|
[209] |
de Abajo F J, Rodríguez-Martín S, Lerma V, et al. Use of renin-angiotensin-aldosterone system inhibitors and risk of COVID-19 requiring admission to hospital: a case-population study. Lancet, 2020; 395(10238): 1705-1714. doi: 10.1016/S0140-6736(20)31030-8
|
[210] |
Lopes R D, Macedo A V S, de Barros E Silva P G M, , et al. Effect of discontinuing vs continuing angiotensin-converting enzyme inhibitors and Angiotensin Ⅱ receptor blockers on days alive and out of the hospital in patients admitted with COVID-19: a randomized clinical trial. JAMA, 2021; 325(3): 254-264. doi: 10.1001/jama.2020.25864
|
[211] |
Cohen J B, Hanff T C, William P, et al. Continuation versus discontinuation of renin-angiotensin system inhibitors in patients admitted to hospital with COVID-19: a prospective, randomised, open-label trial. Lancet Respir Med, 2021; 9(3): 275-284. doi: 10.1016/S2213-2600(20)30558-0
|
[212] |
Haas E J, Angulo F J, McLaughlin J M, et al. Impact and effectiveness of mRNA BNT162b2 vaccine against SARS-CoV-2 infections and COVID-19 cases, hospitalisations, and deaths following a nationwide vaccination campaign in Israel: an observational study using national surveillance data. Lancet, 2021; 397: 1819-1829. doi: 10.1016/S0140-6736(21)00947-8
|
[213] |
Anderson E J, Rouphael N G, Widge A T, et al. Safety and immunogenicity of SARS-CoV-2 mRNA-1273 vaccine in older adults. N Engl J Med, 2020; 383(25): 2427-2438. doi: 10.1056/NEJMoa2028436
|
[214] |
Walsh E E, Frenck R W Jr, Falsey A R, et al. Safety and immunogenicity of two RNA-Based Covid-19 vaccine candidates. N Engl J Med, 2020; 383(25): 2439-2450. doi: 10.1056/NEJMoa2027906
|
[215] |
Keech C, Albert G, Cho I, et al. Phase 1-2 trial of a SARS-CoV-2 recombinant spike protein nanoparticle vaccine. N Engl J Med, 2020; 383(24): 2320-2332. doi: 10.1056/NEJMoa2026920
|
[216] |
Sadoff J, Gray G, Vandebosch A, et al. Safety and efficacy of single-dose Ad26. COV2. S vaccine against Covid-19. N Engl J Med, 2021; 384: 2187-2201. doi: 10.1056/NEJMoa2101544
|
[217] |
Sadoff J, Le Gars M, Shukarev G, et al. Interim results of a phase 1-2a trial of Ad26. COV2. S Covid-19 vaccine. N Engl J Med, 2021; 384: 1824-1835. doi: 10.1056/NEJMoa2034201
|
[218] |
Polack F P, Thomas S J, Kitchin N, et al. Safety and efficacy of the BNT162b2 mRNA Covid-19 vaccine. N Engl J Med, 2020; 383(27): 2603-2615. doi: 10.1056/NEJMoa2034577
|
[219] |
Baden L R, El Sahly H M, Essink B, et al. Efficacy and safety of the mRNA-1273 SARS-CoV-2 vaccine. N Engl J Med, 2021; 384(5): 403-416. doi: 10.1056/NEJMoa2035389
|
[220] |
Dagan N, Barda N, Kepten E, et al. BNT162b2 mRNA Covid-19 Vaccine in a Nationwide Mass Vaccination Setting. N Engl J Med, 2021; 384(15): 1412-1423. doi: 10.1056/NEJMoa2101765
|
[221] |
Goepfert P A, Fu B, Chabanon A L, et al. Safety and immunogenicity of SARS-CoV-2 recombnant protein vaccine formulations in healthy adults: interim results of a randomised, placebo-controlled, phase 1-2, dose-ranging study. Lancet Infect Dis, 2021; 21: 1257-1270. doi: 10.1016/S1473-3099(21)00147-X
|
[222] |
Stephenson K E, Le Gars M, Sadoff J, et al. Immunogenicity of the Ad26. COV2. S Vaccine for COVID-19. JAMA, 2021; 325(15): 1535-44. doi: 10.1001/jama.2021.3645
|
[223] |
Monin L, Laing A G, Muñoz-Ruiz M, et al. Safety and immunogenicity of one versus two doses of the COVID-19 vaccine BNT162b2 for patients with cancer: interim analysis of a prospective observational study. Lancet Oncol, 2021; 22: 765-778. doi: 10.1016/S1470-2045(21)00213-8
|
[224] |
Folegatti P M, Ewer K J, Aley P K, et al. Safety and immunogenicity of the ChAdOx1 nCoV-19 vaccine against SARS-CoV-2: a preliminary report of a phase 1/2, single-blind, randomised controlled trial. Lancet, 2020; 396(10249): 467-78. doi: 10.1016/S0140-6736(20)31604-4
|
[225] |
Xia S, Zhang Y, Wang Y, et al. Safety and immunogenicity of an inactivated SARS-CoV-2 vaccine, BBIBP-CorV: a randomised, double-blind, placebo-controlled, phase 1/2 trial. Lancet Infect Dis, 2021; 21(1): 39-51. doi: 10.1016/S1473-3099(20)30831-8
|
[226] |
Zhang Y, Zeng G, Pan H, et al. Safety, tolerability, and immunogenicity of an inactivated SARS-CoV-2 vaccine in healthy adults aged 18-59 years: a randomised, double-blind, placebo-controlled, phase 1/2 clinical trial. Lancet Infect Dis, 2021; 21(2): 181-192. doi: 10.1016/S1473-3099(20)30843-4
|
[227] |
Zhu F C, Guan X H, Li Y H, et al. Immunogenicity and safety of a recombinant adenovirus type-5-vectored COVID-19 vaccine in healthy adults aged 18 years or older: a randomised, double-blind, placebo-controlled, phase 2 trial. Lancet, 2020; 396(10249): 479-488. doi: 10.1016/S0140-6736(20)31605-6
|
[228] |
Logunov D Y, Dolzhikova I V, Shcheblyakov D V, et al. Safety and efficacy of an rAd26 and rAd5 vector-based heterologous prime-boost COVID-19 vaccine: an interim analysis of a randomised controlled phase 3 trial in Russia. Lancet, 2021; 397(10275): 671-681. doi: 10.1016/S0140-6736(21)00234-8
|
[229] |
Ramasamy M N, Minassian A M, Ewer K J, et al. Safety and immunogenicity of ChAdOx1 nCoV-19 vaccine administered in a prime-boost regimen in young and old adults (COV002): a single-blind, randomised, controlled, phase 2/3 trial. Lancet, 2021; 396(10267): 1979-1993.
|
[230] |
Voysey M, Clemens S A C, Madhi S A, et al. Safety and efficacy of the ChAdOx1 nCoV-19 vaccine (AZD1222) against SARS-CoV-2: an interim analysis of four randomised controlled trials in Brazil, South Africa, and the UK. Lancet, 2021; 397(10269): 99-111. doi: 10.1016/S0140-6736(20)32661-1
|
[231] |
Voysey M, Costa Clemens S A, Madhi S A, et al. Single-dose administration and the influence of the timing of the booster dose on immunogenicity and efficacy of ChAdOx1 nCoV-19 (AZD1222) vaccine: a pooled analysis of four randomised trials. Lancet, 2021; 397(10277): 881-891. doi: 10.1016/S0140-6736(21)00432-3
|
[232] |
Ella R, Vadrevu K M, Jogdand H, et al. Safety and immunogenicity of an inactivated SARS-CoV-2 vaccine, BBV152: a double-blind, randomised, phase 1 trial. Lancet Infect Dis, 2021; 21(5): 637-646. doi: 10.1016/S1473-3099(20)30942-7
|
[233] |
Richmond P, Hatchuel L, Dong M, et al. Safety and immunogenicity of S-Trimer (SCB-2019), a protein subunit vaccine candidate for COVID-19 in healthy adults: a phase 1, randomised, double-blind, placebo-controlled trial. Lancet, 2021; 397(10275): 682-694. doi: 10.1016/S0140-6736(21)00241-5
|
[234] |
Chappell K J, Mordant F L, Li Z, et al. Safety and immunogenicity of an MF59-adjuvanted spike glycoprotein-clamp vaccine for SARS-CoV-2: a randomised, double-blind, placebo-controlled, phase 1 trial. Lancet Infect Dis, 2021; 21: 1383-1394. doi: 10.1016/S1473-3099(21)00200-0
|
[235] |
Xia S, Duan K, Zhang Y, et al. Effect of an inactivated vaccine against SARS-CoV-2 on safety and immunogenicity outcomes: interim analysis of 2 randomized clinical trials. JAMA, 2020; 324(10): 951-960. doi: 10.1001/jama.2020.15543
|
[236] |
Wu Z, Hu Y, Xu M, et al. Safety, tolerability, and immunogenicity of an inactivated SARS-CoV-2 vaccine (CoronaVac) in healthy adults aged 60 years and older: a randomised, double-blind, placebo-controlled, phase 1/2 clinical trial. Lancet Infect Dis, 2021; 21: 803-812. doi: 10.1016/S1473-3099(20)30987-7
|
[237] |
Greinacher A, Thiele T, Warkentin T E, et al. Thrombotic thrombocytopenia after ChAdOx1 nCov-19 vaccination. N Engl J Med, 2021.
|
[238] |
Schultz N H, Sørvoll I H, Michelsen A E, et al. Thrombosis and thrombocytopenia after ChAdOx1 nCoV-19 vaccination. N Engl J Med, 2021.
|
[239] |
Scully M, Singh D, Lown R, et al. Pathologic antibodies to platelet factor 4 after ChAdOx1 nCoV-19 vaccination. N Engl J Med, 2021; 384: 2202-2211. doi: 10.1056/NEJMoa2105385
|
[240] |
Muir K L, Kallam A, Koepsell S A, et al. Thrombotic thrombocytopenia after Ad26. COV2. S vaccination. N Engl J Med, 2021; 384: 1964-1965. doi: 10.1056/NEJMc2105869
|
[241] |
Pottegård A, Lund L C, Karlstad Ø, et al. Events, venous thromboembolism, thrombocytopenia, and bleeding after vaccination with Oxford-AstraZeneca ChAdOx1-S in Denmark and Norway: population based cohort study. BMJ, 2021; 373: n1114.
|
[242] |
Goldberg Y, Mandel M, Bar-On Y M, et al. Waning immunity after the BNT162b2 vaccine in israel. n engl j med, 2021; 385(24): e85. doi: 10.1056/NEJMoa2114228
|
[243] |
Levin E G, Lustig Y, Cohen C, et al. Waning immune humoral response to BNT162b2 Covid-19 vaccine over 6 months. N Engl J Med, 2021; 385(24): e84. doi: 10.1056/NEJMoa2114583
|
[244] |
Atmar R L, Lyke K E, Deming M E, et al. Homologous and heterologous Covid-19 booster vaccinations. N Engl J Med, 2022; 386(11): 1046-1057. doi: 10.1056/NEJMoa2116414
|
[245] |
Stuart A S V, Shaw R H, Liu X, et al. Immunogenicity, safety, and reactogenicity of heterologous COVID-19 primary vaccination incorporating mRNA, viral-vector, and protein-adjuvant vaccines in the UK (Com-COV2): a single-blind, randomised, phase 2, non-inferiority trial. Lancet, 2022; 399(10319): 36-49. doi: 10.1016/S0140-6736(21)02718-5
|
[246] |
Munro A P S, Janani L, Cornelius V, et al. Safety and immunogenicity of seven COVID-19 vaccines as a third dose (booster) following two doses of ChAdOx1 nCov-19 or BNT162b2 in the UK (COV-BOOST): a blinded, multicentre, randomised, controlled, phase 2 trial. Lancet, 2021; 398(10318): 2258-2276. doi: 10.1016/S0140-6736(21)02717-3
|
[247] |
Rearte A, Castelli J M, Rearte R, et al. Effectiveness of rAd26-rAd5, ChAdOx1 nCoV-19, and BBIBP-CorV vaccines for risk of infection with SARS-CoV-2 and death due to COVID-19 in people older than 60 years in Argentina: a test-negative, case-control, and retrospective longitudinal study. Lancet, 2022; 399(10331): 1254-1264. doi: 10.1016/S0140-6736(22)00011-3
|
[248] |
Costa Clemens S A, Weckx L, Clemens R, et al. Heterologous versus homologous COVID-19 booster vaccination in previous recipients of two doses of CoronaVac COVID-19 vaccine in Brazil (RHH-001): a phase 4, non-inferiority, single blind, randomised study. Lancet, 2022; 399(10324): 521-529. doi: 10.1016/S0140-6736(22)00094-0
|
[249] |
Widge A T, Rouphael N G, Jackson L A, et al. Durability of responses after SARS-CoV-2 mRNA-1273 vaccination. N Engl J Med, 2021; 384(1): 80-82. doi: 10.1056/NEJMc2032195
|
[250] |
Doria-Rose N, Suthar M S, Makowski M, et al. Antibody persistence through 6 months after the second dose of mRNA-1273 vaccine for Covid-19. N Engl J Med, 2021; 384: 2259-2261. doi: 10.1056/NEJMc2103916
|
[251] |
Wang Z, Wang Y, Yang Z, et al. The use of non-invasive ventilation in COVID-19: a systematic review. Int J Infect Dis, 2021; 106: 254-261. doi: 10.1016/j.ijid.2021.03.078
|
[252] |
Schünemann H J, Khabsa J, Solo K, et al. Ventilation techniques and risk for transmission of Coronavirus disease, including COVID-19: a living systematic review of multiple streams of evidence. Ann Intern Med, 2020; 173(3): 204-216. doi: 10.7326/M20-2306
|
[253] |
Grieco D L, Menga L S, Cesarano M, et al. Effect of helmet noninvasive ventilation vs high-flow nasal oxygen on days free of respiratory support in patients with COVID-19 and moderate to severe hypoxemic respiratory failure: the HENIVOT randomized clinical trial. JAMA, 2021; 325(17): 1731-1743. doi: 10.1001/jama.2021.4682
|
[254] |
Sartini C, Tresoldi M, Scarpellini P, et al. Respiratory parameters in patients with COVID-19 after using noninvasive ventilation in the prone position outside the intensive care unit. JAMA, 2020; 323(22): 2338-2340. doi: 10.1001/jama.2020.7861
|
[255] |
Coppo A, Bellani G, Winterton D, et al. Feasibility and physiological effects of prone positioning in non-intubated patients with acute respiratory failure due to COVID-19 (PRON-COVID): a prospective cohort study. Lancet Respir Med, 2020; 8(8): 765-774. doi: 10.1016/S2213-2600(20)30268-X
|
[256] |
Botta M, Tsonas A M, Pillay J, et al. Ventilation management and clinical outcomes in invasively ventilated patients with COVID-19 (PRoVENT-COVID): a national, multicentre, observational cohort study. Lancet Respir Med, 2021; 9(2): 139-148. doi: 10.1016/S2213-2600(20)30459-8
|
[257] |
Barbaro R P, MacLaren G, Boonstra P S, et al. Extracorporeal membrane oxygenation support in COVID-19: an international cohort study of the Extracorporeal Life Support Organization registry. Lancet, 2020; 396(10257): 1071-1078. doi: 10.1016/S0140-6736(20)32008-0
|
[258] |
Schmidt M, Hajage D, Lebreton G, et al. Extracorporeal membrane oxygenation for severe acute respiratory distress syndrome associated with COVID-19: a retrospective cohort study. Lancet Respir Med, 2020; 8(11): 1121-1131. doi: 10.1016/S2213-2600(20)30328-3
|
[259] |
Lebreton G, Schmidt M, Ponnaiah M, et al. Extracorporeal membrane oxygenation network organisation and clinical outcomes during the COVID-19 pandemic in Greater Paris, France: a multicentre cohort study. Lancet Respir Med, 2021; 9: 851-862. doi: 10.1016/S2213-2600(21)00096-5
|
[260] |
Bharat A, Machuca T N, Querrey M, et al. Early outcomes after lung transplantation for severe COVID-19: a series of the first consecutive cases from four countries. Lancet Respir Med, 2021; 9(5): 487-497. doi: 10.1016/S2213-2600(21)00077-1
|
[261] |
Morin L, Savale L, Pham T, et al. Four-Month clinical status of a cohort of patients after hospitalization for COVID-19. JAMA, 2021; 325(15): 1525-1534. doi: 10.1001/jama.2021.3331
|
[262] |
Wu X, Liu X, Zhou Y, et al. 3-month, 6-month, 9-month, and 12-month respiratory outcomes in patients following COVID-19-related hospitalisation: a prospective study. Lancet Respir Med, 2021; 9(7): 747-754. doi: 10.1016/S2213-2600(21)00174-0
|
[263] |
Myall K J, Mukherjee B, Castanheira A M, et al. Persistent post-COVID-19 interstitial lung disease. An observational study of corticosteroid treatment. Ann Am Thorac Soc, 2021; 18(5): 799-806. doi: 10.1513/AnnalsATS.202008-1002OC
|
[264] |
Taquet M, Geddes J R, Husain M, et al. 6-month neurological and psychiatric outcomes in 236 379 survivors of COVID-19: a retrospective cohort study using electronic health records. Lancet Psych, 2021; 8(5): 416-427. doi: 10.1016/S2215-0366(21)00084-5
|
[265] |
Ayoubkhani D, Khunti K, Nafilyan V, et al. Post-covid syndrome in individuals admitted to hospital with Covid-19: retrospective cohort study. BMJ, 2021; 372: n693.
|
[266] |
Aldhahir A M, Aldabayan Y S, Alqahtani J S, et al. A double-blind randomised controlled trial of protein supplementation to enhance exercise capacity in COPD during pulmonary rehabilitation: a pilot study. ERJ Open Res, 2021; 7(1): 747-754.
|
[267] |
Center for Global Development. Financing for Global Health Security and Pandemic Preparedness: Taking Stock and What's Next.
|
[1] | Siyu Hao, Yu Zhang, Anqi Yin, Ying Lyu, Nannan Tong, Jiangtian Tian, Yuzhen Li. Toll-like receptors 2 polymorphism is associated with psoriasis: A case-control study in the northern Chinese population[J]. Frigid Zone Medicine, 2024, 4(2): 96-101. doi: 10.1515/fzm-2024-0010 |
[2] | Wen Li, Jia Wang, Yilian Yang, Chunlei Duan, Bing Shao, Mingxiu Zhang, Jiapan Wang, Peifeng Li, Ye Yuan, Yan Zhang, Hongyu Ji, Xingda Li, Zhimin Du. Ethanol extract of cassia seed alleviates metabolic dysfunction-associated steatotic liver disease by acting on multiple lipid metabolism-related pathways[J]. Frigid Zone Medicine, 2024, 4(3): 160-176. doi: 10.1515/fzm-2024-0017 |
[3] | Jiayue Zhang, Liyao Sun, Xiaohan Yu, Chen Yang, Qi An, Chaoqun Wei, Hongyan Ge. LCN2 aggravates diabetic cataracts by promoting ferroptosis in lens epithelial cells[J]. Frigid Zone Medicine, 2024, 4(3): 177-192. doi: 10.1515/fzm-2024-0018 |
[4] | Cheng Wang, Zican Li, Dongwei Guan, Hongxin Fu, Rennan Feng. Seasonal variation in dietary intake and its association with obesity-related chronic diseases in northeast China[J]. Frigid Zone Medicine, 2024, 4(3): 129-136. doi: 10.1515/fzm-2024-0014 |
[5] | Yanying Wang, Jian Huang, Han Sun, Jie Liu, Yingchun Shao, Manyu Gong, Xuewen Yang, Dongping Liu, Zhuo Wang, Haodong Li, Yanwei Zhang, Xiyang Zhang, Zhiyuan Du, Xiaoping Leng, Lei Jiao, Ying Zhang. Long non-coding RNA-AK138945 regulates myocardial ischemia-reperfusion injury via the miR-1-GRP94 signaling pathway[J]. Frigid Zone Medicine, 2024, 4(1): 31-40. doi: 10.2478/fzm-2024-0004 |
[6] | Lina Xuan, Huishan Luo, Shu Wang, Guangze Wang, Xingmei Yang, Jun Chen, Jianjun Guo, Xiaomeng Duan, Xiufang Li, Hua Yang, Shengjie Wang, Hailong Zhang, Qingqing Zhang, Shulei Liu, Yongtao She, Kai Kang, Lihua Sun. Circulating CCRR serves as potential novel biomarker for predicting acute myocardial infarction[J]. Frigid Zone Medicine, 2024, 4(3): 137-151. doi: 10.1515/fzm-2024-0015 |
[7] | Tongzhu Jin, Zhen Ye, Ruonan Fang, Yue Li, Wei Su, Qianqian Wang, Tianyu Li, Hongli Shan, Yanjie Lu, Haihai Liang. Notum protects against myocardial infarction-induced heart dysfunction by alleviating cardiac fibrosis[J]. Frigid Zone Medicine, 2024, 4(1): 41-50. doi: 10.2478/fzm-2024-0005 |
[8] | Yanan Ni, Dan Liu, Xiaona Zhang, Hong Qiao. Association of point in range with β-cell function and insulin sensitivity of type 2 diabetes mellitus in cold areas[J]. Frigid Zone Medicine, 2023, 3(4): 242-252. doi: 10.2478/fzm-2023-0031 |
[9] | Manyu Gong, Xuewen Yang, Yaqi Wang, Yanying Wang, Dongping Liu, Haodong Li, Yunmeng Qu, Xiyang Zhang, Yanwei Zhang, Han Sun, Lei Jiao, Ying Zhang. Growth differentiation factor 11 promotes macrophage polarization towards M2 to attenuate myocardial infarction via inhibiting Notch1 signaling pathway[J]. Frigid Zone Medicine, 2023, 3(1): 53-64. doi: 10.2478/fzm-2023-0008 |
[10] | Yanbin Chu, Rong Wang. Present situation of rational drug use in plateau area[J]. Frigid Zone Medicine, 2023, 3(2): 92-96. doi: 10.2478/fzm-2023-0012 |
[11] | Xin Zhao, Lihua Sun, Chao Chen, Jieru Xin, Yan Zhang, Yunlong Bai, Zhenwei Pan, Yong Zhang, Baoxin Li, Yanjie Lv, Baofeng Yang. Hydroxychloroquine induces long QT syndrome by blocking hERG channel[J]. Frigid Zone Medicine, 2023, 3(2): 105-113. doi: 10.2478/fzm-2023-0014 |
[12] | Yulu Zheng, Zheng Guo, Zhiyuan Wu, Jun Wen, Haifeng Hou. Comparisons of different statistical models for analyzing the effects of meteorological factors on COVID-19[J]. Frigid Zone Medicine, 2023, 3(3): 161-166. doi: 10.2478/fzm-2023-0020 |
[13] | Yongting Zhao, Xiaofang Zhang, Haihai Liang, Lihong Wang. Antidiabetic agents: Do they hit the right targets?[J]. Frigid Zone Medicine, 2022, 2(4): 225-243. doi: 10.2478/fzm-2022-0030 |
[14] | Jie Yang, Zhuxiao Ren, Lingkong Zeng, Shiwen Xia, Lin Wang, Jiayu Miao, Zhe Zhao, Chuanzhong Yang, Xiuyong Cheng, Huayan Zhang, Yuanfang Zhu, Li Liu, Xirong Gao, Bin Yi, Zhenlang Lin, Wei Liu, Xiaoyu Zhou, Benqing Wu, Ling Chen, Zhankui Li, Xuefeng Zhang, Wei Lu, Lianhong Zhang, Xiao Chen, Xiaoyun Zhong, Falin Xu, Jinhui Wu, Yong Ji, Jiahua Pan, Yanxiang Chen, Carine Ronsmans, Zhichun Feng, on behalf of the Chinese Neonatologist Association. Challenges and improvement in management of neonates born to mothers with COVID-19 in China[J]. Frigid Zone Medicine, 2022, 2(2): 94-102. doi: 10.2478/fzm-2022-0013 |
[15] | Baofeng Yang. Inaugural Editorial[J]. Frigid Zone Medicine, 2021, 1(1): 1-1. doi: 10.2478/fzm-2021-0001 |
[16] | Yan Feng, Ying Li, Xinling Yang, Limin Han, Luning Wang, Shan Gao, Ruixue Yin, Xue Wang, Jiayang Li, Meiming Liu, Baiyan Li. Direct evidence of VEGF-mediated neuroregulation and afferent explanation of blood pressure dysregulation during angiogenic therapy[J]. Frigid Zone Medicine, 2021, 1(2): 119-126. doi: 10.2478/fzm-2021-0015 |
[17] | Xin Xing, Shiqiang Wang. Mammalian hibernation: a unique model for medical research[J]. Frigid Zone Medicine, 2021, 1(2): 65-68. doi: 10.2478/fzm-2021-0008 |
[18] | De-an Guo, Wenlong Wei, Changliang Yao, Jianqing Zhang, Qirui Bi. Chinese herbal medicines with beneficial effects[J]. Frigid Zone Medicine, 2021, 1(2): 79-83. doi: 10.2478/fzm-2021-0010 |
[19] | Yanyan Liu, Yahan Yu, Xinyao Wang, Guanqun Liu, Xinda Yin, Yunlong Bai, Zhimin Du. Overexpression of microRNA-135b-5p attenuates acute myocardial infarction injury through its antioxidant and anti-apptotic properties[J]. Frigid Zone Medicine, 2021, 1(2): 85-94. doi: 10.2478/fzm-2021-0011 |
[20] | Qi Wang, Zhouqiao Wu, Jinyao Shi, Kan Xue, Ziyu Li, Jiafu Ji. Utilizing local anti-cancer treatment and online medical service during the COVID-19 pandemic[J]. Frigid Zone Medicine, 2021, 1(2): 127-128. doi: 10.2478/fzm-2021-0016 |
Drug | Enrollment period | The main countries | No. randomized | Dosing scheme | Control | Primary endpoint | Primary endpoint met | Main findings | Main adverse events |
Lopinavir-ritonavir | Feb 10 - Mar 20, 2020 | China | 127 | Ribavirin 400 mg every 12 h, three doses of 8 million international units of interferon beta-1b on alternate days and Lopinavir 400 mg + ritonavir 100 mg twice daily for 14 days | Lopinavir 400 mg + ritonavir 100 mg twice daily for 14 days | Time to nasopharyngeal swab negative for SARS-CoV-2 | Yes | The combination group had a significantly shorter median time from start of study treatment to negative nasopharyngeal swab | Nausea, diarrhea |
Jan 18 - Feb 3, 2020 | China | 199 | Lopinavir 400 mg + ritonavir 100 mg twice daily for 14 days | Standard care | Time to clinical improvement | No | No difference in the time to clinical improvement, viral loads and mortality at 28 days | Nausea, vomiting, and diarrhea | |
Mar 19 - Jun 29, 2020 | United Kingdom | 5, 040 | Lopinavir 400 mg + ritonavir 100 mg twice daily for 10 days until discharge | Standard care | 28-day all-cause mortality | No | No significant difference in time until discharge alive from hospital or the proportion of patients discharged from hospital alive within 28 days | Not specified | |
Mar - Jul 4, 2020 | 30 countries | 11, 330 (1, 411 in lopinavir group) | Two tablets twice daily for 14 days | The local standard of care | in-hospital mortality | No | Death occurred in 148 of 1, 399 patients receiving lopinavir and in 146 of 1, 372 receiving a control drug (rate ratio, 1.00; 95% CI, 0.79 to 1.25) | Not specified | |
Remdesivir | Feb 21 - Apr 19, 2020 | United States | 1, 062 | Remdesivir 200 mg on day 1, followed by 100 mg daily for up to 9 additional days) | placebo for up to 10 days | Time to clinical recovery | Yes | Patients receiving remdesivir had a significantly shorter median recovery time (10 vs. 15 days) | Not specified |
Mar 15-Apr 18, 2020 | United States, Europe and Asia | 596 | Remdesivir intravenously at 200 mg on day 1 followed by 100 mg/d | Standard care | Clinical status on day 11 on a 7-point ordinal scale | Yes | Patients in the 5-day group had significantly higher odds of a better clinical status distribution | Nausea, hypokalemia, and headache | |
Mar 6 - Mar 26, 2020 | United States and other countries | 397 | Intravenous remdesivir for 5 days | intravenous remdesivir for 10 days | Clinical status on day 14, assessed on a 7-point ordinal scale | No | A clinical improvement of 2 points or more on the ordinal scale occurred in 64% of patients in the 5-day group and in 54% in the 10-day group | Nausea, worsening respiratory failure, elevated alanine aminotransferase level, constipation | |
Feb 6 - Mar 12, 2020 | China | 237 | Remdesivir 200 mg on day 1 followed by 100 mg on days 2-10 in single daily infusions for 10 days | The same volume of placebo infusions for 10 days | Time to a decline of two levels on a six-point ordinal scale of clinical status or discharged from hospital | No | Remdesivir use was not associated with a difference in time to clinical improvement | Constipation, hypoalbuminaemia, hypokalaemia, anaemia, thrombocytopenia, increased total bilirubin | |
Mar 2020 - NA | 30 countries | 11, 330 (2, 750 in remdesivir group) | 200 mg on day 0 and 100 mg intravenously on days 1 through 9 | The local standard of care | in-hospital mortality | No | Death occurred in 301 of 2, 743 patients receiving remdesivir and in 303 of 2, 708 receiving a control agent (rate ratio, 0.95; 95% CI, 0.81 to 1.11) | Not specified | |
Hydroxychloroquine | Mar 25 - Jun 5, 2020 | United Kingdom | 4, 716 | The usual standard of care plus hydroxychloroquine or one of the other available treatments | Usual standard of care | 28-day mortality | No | No significant difference in death within 28 days (27.0% in the hydroxychloroquine group vs. 25.0% in the usual-care group) | Supraventricular tachycardia, atrial flutter |
Mar 29 - May 17, 2020 | Brazil | 667 | Standard care plus hydroxychloroquine at 400 mg twice daily plus azithromycin at 500 mg once daily for 7 days | Standard care; standard care plus hydroxychloroquine at 400 mg twice daily | Clinical status at 15 days as assessed with the use of seven-level ordinal scale | No | The odds of having a higher score at 15 days was not affected by hydroxychloroquine alone (odds ratio: 1.21) or hydroxychloroquine plus azithromycin (odds ratio: 0.99) | Prolongation of the QTc interval, elevated liver enzymes | |
Apr 2 - Jul 17, 2020 | United States | 479 | Hydroxychloroquine (400 mg twice daily for 2 doses, then 200 mg twice daily for 8 doses) | Placebo | Clinical status 14 days after randomization (7-category ordinal scale) | No | Clinical status at 14 days did not significantly differ between the hydroxychloroquine and the placebo groups (median: 6 vs. 6). | QTc interval greater than 500 ms | |
Mar - Jun 19, 2020 | 30 countries | 11, 330 (954 in hydroxychloroquine group) | Four tablets at hour 0, four tablets at hour 6, and starting at hour 12, two tablets twice daily for 10 days | The local standard of care | In-hospital mortality | No | Death occurred in 104 of 947 patients receiving hydroxychloroquine and in 84 of 906 receiving a control agent (rate ratio, 1.19; 95% CI, 0.89 to 1.59) | Not specified | |
Feb 11- Feb 29, 2020 | China | 150 | A loading dose of 1, 200 mg daily for three days followed by a maintenance dose of 800 mg daily (total duration: two or three weeks) | Standard care | Negative conversion by 28 days | No | The probability of negative conversion by 28 days in the standard of care plus hydroxychloroquine group (85.4%%) was similar to that in the standard care group (81.3%) | Diarrhoea being most common; serious adverse events rare | |
Mar 17 - May 6, 2020 | United States and Canada | 821 | Hydroxychloroquine 800 mg once, followed by 600 mg in 6-8 hours, then 600 mg daily for 4 additional days | Placebo | Incidence of lab confirmed Covid-19 or illness compatible with Covid-19 within 14 days | No | The incidence of new illness compatible with Covid-19 did not differ significantly | Nausea, loose stools, abdominal discomfort | |
Mar 17 - Apr 28, 2020 | Spain | 2, 314 | Hydroxychloroquine 800 mg once, followed by 400 mg daily for 6 days | Usual care (no specific therapy) | PCR-confirmed, symptomatic Covid-19 within 14 days | No | Similar incidence of PCR-confirmed, symptomatic Covid-19 in the hydroxychloroquine and usual-care groups (5.7% and 6.2%) | Diarrhea, nausea, abdominal pain, drowsiness, headache | |
Ivermectin | Jul 15 - Dec 21, 2020 | Colombia | 476 | ivermectin 300 μg/kg of body weight per day for 5 days | Placebo for 5 days | Time to resolution of symptoms within 21-day follow-up | No | The median time to resolution of symptoms was 10 days in the ivermectin group compared with 12 days in the placebo group | Headache, dizziness, diarrhea, nausea |
Favipiravir | Feb 4 - Mar 8, 2020 | Iran | 380 | Favipiravir (1.6 gr loading, 1.8 gr daily) | Lopinavir/Ritonavir (800/200 mg daily) | The number of admissions to the intensive care unit | No | The number of deaths, intubations, and ICU admissions were not significantly different | Nausea, anorexia, fatigue, headache |
Interferon beta-1a | Mar 30 - May 30, 2020 | United Kingdom | 101 | 6 MIU interferon beta-1a by inhalation via a mouthpiece daily for 14 days | Placebo | Change in clinical condition on the WHO Ordinal Scale for Clinical Improvement (OSCI) during the dosing period | Yes | Patients receiving SNG001 had greater odds of improvement on the OSCI scale (odds ratio 2.32) on day 15 or 16 and were more likely than those receiving placebo to recover to an OSCI score of 1 (no limitation of activities) during treatment | Headache; few serious adverse events |
Mar - Jul 4, 2020 | 30 countries | 11, 330 (2, 063 in interferon beta-1a group) | Subcutaneous: three doses over 6 days (randomization and days 3 and 6) of 44 μg of interferon beta-1a Intravenous: 10 μg daily for 6 days |
The local standard of care | in-hospital mortality | No | Death occurred in 243 of 2, 050 patients receiving interferon beta-1a and in 216 of 2, 050 receiving its control (rate ratio, 1.16; 95% CI, 0.96 to 1.39) | Not specified | |
Corticosteroids | Mar 19 - Jun 8, 2020 | United Kingdom | 2, 104 | Oral or intravenous dexamethasone (6 mg once daily) for up to 10 days | Usual care | 28-day mortality | Yes | Significantly lower percentage of patients died in the dexamethasone group than in the usual care group (22.9% vs. 25.7%) | Not specified |
Apr 17 - Jun 23, 2020 | Brazil | 299 | Dexamethasone intravenously daily for 5 days, 10 mg dexamethasone daily for 5 days or until ICU discharge, plus standard care | Standard care alone | Ventilator-free days during the first 28 days | Yes | Patients in the dexamethasone group had a mean of 6.6 ventilator-free days vs 4.0 ventilator-free days in the standard care group | Insulin use for glycemia | |
Mar 9 - Jun 17, 2020 | Australia and other countries | 614 | A fixed 7-day course of intravenous hydrocortisone (50 mg or 100 mg every 6 hours) A shock-dependent course (50 mg every 6 hours) | No hydrocortisone | Organ support-free days within 21 days (Bayesian cumulative logistic model) | Yes | The adjusted Bayesian probability of superiority was 93% for the fixed-dose hydrocortisone group | Not specified | |
Mar 7-Jun 1, 202 | France | 149 | Continuous intravenous infusion of hydrocortisone at 200 mg/d at day 1 and continued at 200 mg/d until day 7 and then decreased to 100 mg/d for 4 days and 50 mg/d for 3 days | Placebo (saline) for 14 days | Treatment failure on day 21 | No | No significant difference in the percentage of patients (42.1%) in the hydrocortisone group and in the placebo group (50.7%), who reached the primary endpoint | Not specified | |
Azithromycin | Apr 7 - Nov 27, 2020 | United Kingdom | 16, 442 | Usual standard of care plus azithromycin 500 mg once per day by mouth or intravenously for 10 days or until discharge | Usual standard of care alone for 10 days or until discharge | 28-day all-cause mortality | No | No significant difference in the 28-day all-cause mortality between the azithromycin group and the control group (22% vs. 22%) | Not specified |
May 22 - Nov 30, 2020 | United Kingdom | 2, 265 | Usual care plus azithromycin 500 mg daily for three days | Usual care plus other interventions, or usual care alone | Time to first recovery in 28 days Hospital admission or death related to COVID-19 in 28 days |
No | No significant effect by azithromycin in time to first reported recovery; No significant difference in the percentage of participants being hospitalized or died | Not specified | |
Mar 28 - May 19, 2020 | Brazil | 447 | Azithromycin (500 mg via oral, asogastric, or intravenous administration once daily for 10 days) plus standard of care | Standard of care without macrolides | SARS-CoV-2 infection confirmed by testing before randomisation | No | SARS-CoV-2 infection was not significantly different between the azithromycin and control groups (odds ratio: 1.36) | QTc interval prolongation, gastrointestinal intolerance | |
Baricitinib | May 8- Jul 1, 2020 | United States | 1, 033 | Remdesivir: 200-mg on day 1, 100-mg daily on days 2-10 or until hospital discharge or death Baricitinib: 4-mg daily dose for 14 days or until hospital discharge |
Placebo | Time to recovery within 15 days | Yes | Patients receiving baricitinib experienced a shorter time to recovery and had higher odds of improvement in clinical status at day 15 | Hyperglycemia, anemia, decreased lymphocyte count, acute kidney injury |
Fluvoxamine | Apr 10 - Aug 5, 2020 | United States | 181 | 100 mg of fluvoxamine 3 times daily for 15 days | Placebo 3 times daily for 15 days | Clinical deterioration within 15 days of randomization | Yes | Clinical deterioration occurred in 0 of 80 patients in the fluvoxamine group and in 6 of 72 patients in the placebo group | Pneumonia, nausea, vomiting |
Inhaled budesonide | Jul 16 - Dec 9, 2020 | United Kingdom | 146 | Budesonide dry powder 800 μg twice daily until symptom resolution | Usual care | COVID-19-related urgent care visit | Yes | The primary outcome occurred in 15% participants in the usual care group and 3% in the budesonide group | Sore throat; dizziness |
Ruxolitinib | Feb 9 - Feb 28, 2020 | China | 43 | Ruxolitinib 5 mg twice a day plus standard-of-care treatment | Placebo (100 mg vitamin C) twice daily with standard care | Time to clinical improvement | No | No differences in terms of clinical improvement between ruxolitinib (median: 12 days) and placebo group (median: 15 days) | Anemia, thrombocytopenia, hypercholestrolemia |
Vitamin D | Jun 2 - Aug 27, 2020 | Brazil | 240 | A single oral dose of 200 000 IU of vitamin D3 | A single dose of placebo | Length of stay | No | Median length of stay was not significantly different between the vitamin D3 (7.0 days) and placebo groups (7.0 days) | Not specified |
Drug | Enrollment period | The main countries | No. randomized | Dosing scheme | Control | Primary endpoint | Primary endpoint met | Main findings | Main adverse events |
Tocilizumab | Mar 9 - Nov 19, 2020 | United Kingdom | 755 | Tocilizumab (8 mg per kilogram of body weight) | Standard care alone | Respiratory and cardiovascular organ support-free days to day 21 | Yes | The median number of organ support-free days was 10 in tocilizumab group and 0 in control group (median adjusted cumulative odds ratios: 1.64) | Not specified |
Apr 23, 2020, - Jan 24, 2021 | United Kingdom | 4, 116 | Tocilizumab 400 mg-800 mg given intravenously (the 2nd dose can be given 12-24 h later) plus standard of care | Standard of care alone | 28-day mortality | Yes | 31% of patients allocated tocilizumab and 35% of patients allocated to usual care died within 28 days (rate ratio 0·85; 95% CI 0·76-0·94) | Not specified | |
Apr 20 - Jun 15, 2020 | United States | 243 | Tocilizumab (8 mg per kilogram of body weight administered intravenously, not to exceed 800 mg) | Placebo | Intubation or death, assessed in a time-to-event analysis | No | The hazard ratio for intubation or death in the tocilizumab group compared with the placebo group was 0.83 (95% confidence interval: 0.38 - 1.81) | Neutropenia, elevated liver enzyme | |
Apr 3 - May 28, 2020 | Europe and North America | 452 | A single intravenous infusion of tocilizumab (at a dose of 8 mg per kilogram of body weight) | Placebo intravenous injection | Clinical status at day 28 on an ordinal scale ranging from 1 to 7 | No | The median value for clinical status at day 28 was 1.0 in the tocilizumab group and 2.0 in the placebo group | Infection, bleeding, hypersensitivity | |
May 30 - Aug 31, 2020 | India | 180 | Tocilizumab 6 mg/kg plus standard care | Standard care alone | Progression of COVID-19 up to day 14 | No | Progression of COVID-19 occurred in 9% of patients in the tocilizumab group and 13% in the standard care group (difference −3·71; p=0·42) | Infections | |
Mar 31 - Apr 18, 2020 | France | 131 | Tocilizumb 8 mg/kg, intravenously plus usual care on day 1 and on day 3 if clinically indicated | Usual care alone | Scores higher than 5 on WHO 10-point Clinical Progression Scale on day 4 and survival without ventilation at day 14 | No | In the tocilizumab group, 12 patients vs 19 in the usual care group had scores higher than 5. At day 14, 12% fewer patients needed ventilation or died in the tocilizumab group. | Hepatic cytolysis, anemia, neutropenia | |
Mar 31 - Jun 11, 2020 | Italy | 126 | Intravenous tocilizumab within 8 hours from randomization (8 mg/kg up to 800 mg), followed by a second dose after 12 hours | Supportive care until clinical worsening (could receive tocilizumab) | Entry into intensive care unit with invasive ventilation, death from all causes, or clinical aggravation | No | 28.3% in tocilizumab arm and 27.0% in the standard care group showed clinical worsening within 14 days. Two patients in the tocilzumab group and 1 in the control group died before 30 days; 6 and 5 patients were intubated | Laboratory abnormalities, infection, infestation | |
May 8- Jul 20, 2020 | Brazil | 129 | Tocilizumab (single intravenous infusion of 8 mg/kg) plus standard care | standard care alone | Clinical status measured at 15 days | No | 28% of patients in the tocilizumab group and 20% in the standard care group were receiving mechanical ventilation or died at day 15 (odds ratio, 1.54, P=0.32) | Abnormal liver function, anemia, thrombocytopenia | |
Sarilumab | Jun 20 - Nov 19, 2020 | United Kingdom | 755 | Sarilumab (400 mg) | Standard care alone | Respiratory and cardiovascular organ support-free days up to day 21 | Yes | The median of organ support-free days was 11 in the sarilumab group and 0 in the control group | Not specified |
Mar 28 - Jul 3, 2020 | Argentina and other countries | 431 | intravenous sarilumab 400 mg, sarilumab 200 mg | Placebo | Time to clinical improvement of two or more points (seven point scale) | No | No significant differences in median time to improvement between the placebo (12.0 days) and sarilumab 200 mg (10.0 days) or 400 mg groups (10.0 days) | Increased alanine aminotransferase, invasive bacterial or fungal infection | |
Anakinra | Apr 8- Apr 26, 2020 | France | 116 | Usual care plus anakinra (200 mg twice daily on days 1-3, 100 mg twice on day 4, 100 mg once on day 5) | Usual care alone | Proportion of patients who had died or needed ventilation by day 4; Survival without ventilation at day 14 | No | 36% of patients in the anakinra group had WHO-CPS score > 5 at day 4 versus 38% in the usual care group; 47% patients in the anakinra group and 51% in the usual care group needed ventilation or died |
Acute respiratory distress syndrome, bacterial sepsis, hepatic cytolysis |
Granulocyte colony stimulating factor | Feb 18 - Apr 10, 2020 | China | 199 | 3 doses of recombinant human granulocyte colony-stimulating factor (5 μg/kg, subcutaneously at days 0-2) | Standard care | The time to improvement of at least 1 point on 7-category severity score | No | Time to clinical improvement was similar (median 12 days in the rhG-CSF group vs 13 days in the usual care group; hazard ratio, 1.28; 95% CI, 0.95-1.71) | Neutrophilia, osteodynia, muscle soreness |
Marilimumab | May 28 - Sep 15, 2020 | United States | 40 | Mavrilimumab 6 mg/kg as a single intravenous infusion | Placebo intravenous infusion | The proportion of patients alive and off supplemental oxygen therapy at day 14 | No | Similar percentages of patients (odds Ratio: 1·48, 95% confidence interval: 0·43-5·16) remained alive and were off supplemental oxygen therapy | Bacterial pneumonia |
Vilobelimab | Mar 31 - Apr 24, 2020 | The Netherlands | 30 | Vilobelimab (up to seven doses of 800 mg intravenously) plus best supportive care | Best supportive care | Percentage change in PaO2/FiO2 in supine position between baseline and day 5 | No | No differences between treatment groups (17% change in the vilobelimab group vs 41% in the control group; difference 24% [95% CI: 58 to 9]). | pulmonary embolisms; infections |
Vaccine | Enrollment period | The main countries | Participants | No. randomized | Dosing scheme | Control | Primary endpoint | Vaccine efficacy | Efficacy against variants | Main immunogenicity outcomes | Main safety outcomes |
mRNA-1273 | Apr 16 - May 12, 2020 | United States | Older adults | 40 | Two doses of either 25 μg or 100 μg of vaccine administered 28 days apart | NA | Antibody responses on days 1, 15, 29, 36, 43, and 57 | NA | NA | Anti-S-2P GMT: 25-μg: 323, 945 in 56-70 yr; 1, 128, 391 in ≥71 yr group; 100-μg: 1, 183, 066 and 3, 638, 522 | Predominantly mild or moderate in severity (fatigue, chills, headache, myalgia, pain) |
Jul 27 - Oct 23, 2020 | United States | Individuals 18 years of age or older | 30, 420 | A two-dose regimen containing 0.5 mL injections containing 100 μg of mRNA-1273 | Placebo (saline) | Prevention of Covid-19 with onset > 14 days after 2nd injection in non-infected participants | 94.1% | NA | NA | (Mainly) pain after injection | |
BNT162b1 | May 4 - Jun 22, 2020 | United States | Healthy adults (18-55 yrs; 65-85 yrs) | 195 | Two doses of vaccine (10 μg, 20 μg, 30 μg, and 100 μg), with a 21-day interval; One dose 100 μg of BNT162b1 in one group only | Placebo | Safety ( e.g. , local and systemic reactions and adverse events) | NA | NA | Similar dose-dependent neutralizing GMTs in younger and older adults | Lower incidence and severity of systemic reactions than BNT162b2 |
BNT162b2 | Jul 27, - Nov 14, 2020 | United States and other countries | Adults 16 yrs or older | 43, 548 | BNT162b2 vaccine twice (30 μg per dose), at 21-day interval | Placebo | Efficacy against laboratory-confirmed Covid-19 and safety | 95% | NA | NA | Short-term, mild-to-moderate pain at injection site, fatigue, headache |
Dec 20, 2020, to Feb 1, 2021 | Israel | Non-infected individuals aged 16 yrs or greater | 596, 618 | Having received BNT162b2 vaccination | No vaccination | COVID-19 infection, symptomatic Covid-19, hospitalization, severe illness, death | 7 days after 2nd dose: 92% | NA | NA | NA | |
Dec 8 - Dec 29, 2020 | United Kingdom | Patients with cancer | 151 | Two 30 μg doses of BNT162b2 administered intramuscularly 21 days apart | Single dose of vaccine | Seroconversion to spike protein and vaccine boosting after 21 days on seroconversion | NA | NA | One dose yields poor efficacy. Immunogenicity increased in patients with solid cancer in 2 weeks of boost | No toxicities in 54% patients following the first dose | |
Ad26.COV2.S | Jul 22 - Aug 7, 2020 | Belgium and the United States | Healthy adults aged 18-55 yrs and 65 yrs or greater | 805 | 5×1010 viral particles (low dose); 1×1011 viral particles (high dose) per milliliter |
Placebo | Safety and reactogenicity | NA | NA | Neutralizing antibody detected in ≥90% of participants on day 29 after dose 1 and was 100% by day 57 | NA |
Sep 21, 2020 - Jan 22, 2021 | Argentina, Brazil and other countries | Adults 18-59 yrs and 60 yrs or older | 19, 630 | 5×1010 viral particles as a single intramuscular injection (0.5 mL) | Placebo | Vaccine efficacy against moderate to severe-critical Covid-19 with an onset at least 14 and 28 days | 76.7% at ≥14 days; 85.4% at ≥28 days | 52.0% and 64.0% (20H/501Y. V2 variant) | Higher reactogenicity than with placebo (mild to moderate and transient) | injection-site pain, headache, fatigue, myalgia, nausea | |
Jun 18 - Aug 3, 2020 | Russia | Adults aged 18-60 yrs | 76 | One dose of rAd26-S or one dose of rAd5-S | NA | SARS-CoV-2-specific antibodies on days 0, 14, 21, 28, and 42; number of participants with adverse events | NA | NA | At day 42, RBD IgG titers were 14703 (frozen) and 11 143 (lyophilised) Neutralising antibodies: 49·25 and 45·95, respectively | Common: pain at injection site, hyperthermia, headache, asthenia, muscle and joint pain | |
Jul 29 - Aug 7, 2020 | United States | Participants 18-55 yrs old | 25 | 1 or 2 intramuscular injections with 5 × 1010 viral particles or 1 × 1011 viral particles of Ad26. COV2.S vaccine | Placebo | Cellular immune responses and T cell responses | NA | NA | Binding and neutralizing antibodies emerged by day 8 in 90% and 25% of vaccine recipients | NA | |
Recombinant spike protein nanoparticle vaccine | May 26, - Jun 6, 2020 | Australia | Young and middle-aged adults | 131 | rSARS-CoV-2 vaccine (in 5-μg and 25-μg doses, with or without Matrix-M1 adjuvant) | Placebo | Reactogenicity; serum chemistry, hematology; IgG anti-spike protein response | NA | NA | Two-dose 5-μg regimen induced marked anti-spike IgG and neutralization responses | No serious adverse events; Overall reactogenicity was absent or mild |
Aug 17 - Nov 25, 2020 | South Africa | HIV-positive and -negative adults aged 18-64 yrs | 4, 387 | NVX-CoV2373 (5 μg of recombinant spike protein with 50 μg of Matrix-M1 adjuvant) | Placebo (0.5 mL) | Safety and vaccine efficacy against lab-confirmed symptomatic Covid-19 at 7 days or more after 2nd dose | 60.1% | 51.0% | Vaccine efficacy among HIV-negative participants was 60.1% | Headache, muscle pain, and fatigue most common; no serious adverse events | |
Recombinant spike protein vaccine | Sep 3 - Sep 29, 2020 | Untied States | Adults 18-49 yrs and ≥50 yrs | 441 | One dose (on day 1) or two doses (on days 1 and 22) of placebo or candidate vaccine, containing low dose (effective dose 1·3 μg) or high-dose (2·6 μg) antigen with adjuvant AF03 or AS03 | Unadjuvanted high-dose antigen | Safety up to day 43, and immunogenicity (neutralising antibodies on days 1, 22, and 36) | NA | NA | Neutralizing antibody titers: 13·1 in low-dose + AF03 group, 20·5 in low-dose + AS03 group, 43·2 in high-dose + AF03 group, 75·1 in high-dose + AS03 group | No vaccine-related unsolicited immediate adverse events, serious adverse events |
ChAdOx1 nCoV-19 vaccine | Jun 24 - Nov 9, 2020 | South Africa | Adults 18-65 yrs | 2, 026 | Two doses of vaccine containing 5×1010 viral particles 21 to 35 days apart | Placebo (0.9% saline) | Safety and efficacy against lab-confirmed symptomatic Covid-19 > 14 days after 2nd dose | 21.9% | 21.9% | Strong neutralizing antibodies at 28 days after dose 1 and increased after dose 2 | No serious adverse events |
Apr 23 - May 21, 020 | United Kingdom | Healthy adults aged 18-55 years | 1, 077 | ChAdOx1 nCoV-19 vaccine (5×1010 viral particles) | Menigococcal ACWY vaccine | Cases of symptomatic virologically confirmed COVID-19; Occurrence of serious adverse events |
NA | NA | Anti-spike IgG responses rose by day 28, and were boosted following a second dose | Pain, feverish, chills, muscle ache, headache, malaise; No serious adverse events |
|
May 30 - Aug 8, 2020 | United Kingdom | Adults aged 18-55 yrs, 56-69 yrs, and 70 yrs and older | 560 | intramuscular ChAdOx1 nCoV-19 (2.2×1010 virus particles) | Meningococcal ACWY vaccine | The number of cases of symptomatic, virologically confirmed COVID-19, serious adverse events | NA | NA | Median anti-spike IgG responses 28 days after the boost dose were similar across the three age cohorts | Injection-site pain, feeling feverish, muscle ache, headache (less common in older adults) | |
Apr 23 - Nov 4, 2020 | United Kingdom, Brazil, South Africa | Adults aged 18 yrs and older | 23, 848 | Two doses containing 5×1010 viral particles; A half dose as their first dose; A standard dose as their second dose |
Meningococcal ACWY vaccine | Symptomatic COVID-19 in seronegative participants with PCR-positive swab > 14 days after a second dose | 70.4% | NA | Two standard doses: 62.1%; Low dose followed by standard dose: 90.0% |
Good safety profile with serious adverse events balanced across the study arms | |
Apr 23 - Dec 6, 2020 | United Kingdom, Brazil | individuals aged 18 years and older | 24, 422 | Two standard doses of ChAdOx1 nCoV-19 (5×1010 viral particles) | Control vaccine or placebo | Virologically confirmed symptomatic COVID-19 disease | 66.7% | NA | Antibody levels were maintained with minimal waning by day 90 (geometric mean ratio 0·66 [95%CI 0·59-0·74]) | The most common serious adverse events were infections and infestations | |
May 31 - Dec 30, 2020 | United Kingdom | Healthy people aged 18 years and older | 8, 534 | Standard-dose ChAdOx1 nCoV-19 vaccine (5×1010 viral particles) | Meningococcal conjugate control vaccine | Symptomatic COVID-19 in seronegative participants with positive swab > 14 days after dose 2 | 81.5% for non-B.1.1.7 variant | 70.4% for B.1.1.7 variant | NA | NA | |
BBIBP-CorV | Phase 1: Apr 29 - Jun 28, 2020 Phase 2: May 18 - Jul 30, 2020 |
China | Healthy people Phase 1: 18-80 yrs Phase 2: 18-59 yrs |
640 | A single-dose schedule of 8 μg on day 0; A two-dose schedule of 4 μg on days 0 and 14, 0 and 21, or 0 and 28 |
Placebo | Safety and tolerability | NA | NA | Neutralizing antibody titers on day 28 were greater in the 4 μg days 0 and 14, days 0 and 21, and days 0 and 28 schedules | Mild or moderate; No serious adverse event within 28 days |
Inactivated vaccine | Apr 16 - Apr 25, 020 | China | Adults aged 18-59 years | Phase 1: 144 Phase 2: 743 |
Low dose CoronaVac [3 μg per 0·5 mL of aluminium hydroxide diluent per dose]; High-dose Coronavc [6 μg per 0·5 mL of aluminium hydroxide diluent per dse] |
Placebo | Adverse reactions within 28 days; Seroconversion rates of neutralizing antibodies at day 14 and 28 | NA | NA | Phase 1: Seroconversion rates: 46% in 3μg group, 50% in 6μg group, 0% in placebo group Phase 2: 92%, 98% and 3% |
Phase 1: 29% in the 3μg group, 38% in 6μg group, 8% in the placebo group Phase 2: 33%, 35% and 22% |
July 13 - Jul 30, 2020 | United Kingdom and Brazil | Healthy adults aged 18-55 years | 827 | 3 μg with Algel-IMDG, 6 μg with Algel-IMDG, or 6 μg with Algel | Algel only | Solicited local and systemic reactogenicity events at 2 h and 7 days and throughout the study | NA | NA | Seroconversion rates (%) were 87·9, 91·9, and 82·8 in the 3 μg with Algel-IMDG, 6 μg with Algel-IMDG, and 6 μg with Algel groups | Mild or moderate; more frequent after the first dose | |
May 22 - Jun 1, 2020 | China | healthy adults aged 60 yrs and older | 72 | CoronaVac at 1·5 μg, 3 μg, or 6 μg per dose | Placebo | Adverse reactions within 28; seroconversion rate at 28 days after the second injection | NA | NA | Seroconversion was seen in 90.7% of participants in the 1·5 μg group, 98% in the 3 μg group and 99·0% in the 6 μg group | Mild or moderate in severity; injection site pain was most frequent | |
April 12 - Jul 27, 2020 | China | Healthy adults aged 18-59 yers | Phase 1: 96 Phase 2: 224 |
2.5, 5, and 10 μg/dose inactivated vaccine | Aluminum hydroxide adjuvant | Combined adverse reactions at 7 days Neutralizing antibody response after 14 days | NA | NA | GMTs of neutralizing antibodies in low-, medium-, and high-dose groups at day 14: 316, 206 and 297 in phase 1 | Injection site pain, followed by fever (mild and self-limiting) | |
Non-replicating adenovirus type-5-vectored COVID-19 vaccine | Apr 11 - 16, 2020 | China | Healthy adults aged 18 yrs or older | 603 | 1×1011 viral particles per mL or 5×1010 viral particles per mL | Placebo | GMTs of specific ELISA antibody responses to RBD and neutralising antibody responses at day 28 | NA | NA | The RBD-specific ELISA antibodies peaked at 656·5 and 571·0, with seroconversion rates at 96% and 97% at day 28 | Mostly mild to moderate adverse events; pain being the most common adverse event |
Jun 19 - Sep 23, 2020 | Australia | Younger (18-54 yrs) and older adults (55-75 yrs) | 151 | S-trimer vaccine either 3 μg, 9 μg, or 30 μg | Placebo (0.9% saline) | Safety, tolerability, and immunogenicity of three increasing doses | NA | NA | Fixed doses vaccine induced high titers and seroconversion rates of binding and neutralizing antibodies | Well tolerated overall | |
MF59-adjuvanted subunit vaccine | Jun 23 - Aug 17, 2020 | Australia | Healthy adults (aged ≥18 to ≤55 years) | 314 | Two doses via intramuscular injection 28 days apart of either sclamp vaccine at 5 μg, 15 μg, or 45 μg, or one dose of sclamp vaccine at 45 μg followed by placebo | Placebo | Antigen-specific IgG titer at 28 days; Solicited adverse events in the 7 days Unsolicited events up to 12 months |
NA | NA | Vaccination with SARS-CoV-2 clamp elicited a similar antigen-specific response irrespective of dose | Severe solicited reactions occurred at similar rates in participants receiving vaccines at any dose |
GMT: geometric mean titer; RBD: receptor-binding domain; NAAT: nucleic acid amplification test |
Key unanswered questions related to COVID-19 | Proposed future research directions |
The origin of SARS-CoV-2 and the mode of zoonotic transmissions | To unravel the reservoir of SARS-CoV-2 and understanding the mechanisms of zoonotic infections |
Durability of infectiousness of viral particles in the environment | To investigate the immune escape mechanisms of the emerging variants |
Immunologic mechanisms contributing to the sudden deterioration of clinical status and the clinical recovery | To validate a novel diagnostic method for identifying asymptomatic individuals |
The upper limit of duration of the protective effect of antibodies after natural infection and the receipt of vaccination | To assess the effectiveness of mass vaccination programs in reducing global incidence of COVID-19 |
Factors associated with re-infection | To develop new rapid diagnostic testing for point-of-care use |
Molecular mechanisms underlying prolonged shedding of the live viruses or viral fragments | To identify monoclonal antibody cocktails to improve clinical outcomes |
Association between variants and the efficacy of different types of vaccines | To develop medications that target cellular surface markers in addition to ACE2 |
The mechanisms leading to interstitial lung diseases and the reversibility with immunosuppressants during convalescent phase | To determine the optimal treatment options for non-intubated patients with severe COVID-19 |
The probability and duration of complete recovery after discharge from hospital | To explore new intervention strategies to accelerate the functional and psychological recovery post-discharge from COVID-19 |
Drug | Enrollment period | The main countries | No. randomized | Dosing scheme | Control | Primary endpoint | Primary endpoint met | Main findings | Main adverse events |
Lopinavir-ritonavir | Feb 10 - Mar 20, 2020 | China | 127 | Ribavirin 400 mg every 12 h, three doses of 8 million international units of interferon beta-1b on alternate days and Lopinavir 400 mg + ritonavir 100 mg twice daily for 14 days | Lopinavir 400 mg + ritonavir 100 mg twice daily for 14 days | Time to nasopharyngeal swab negative for SARS-CoV-2 | Yes | The combination group had a significantly shorter median time from start of study treatment to negative nasopharyngeal swab | Nausea, diarrhea |
Jan 18 - Feb 3, 2020 | China | 199 | Lopinavir 400 mg + ritonavir 100 mg twice daily for 14 days | Standard care | Time to clinical improvement | No | No difference in the time to clinical improvement, viral loads and mortality at 28 days | Nausea, vomiting, and diarrhea | |
Mar 19 - Jun 29, 2020 | United Kingdom | 5, 040 | Lopinavir 400 mg + ritonavir 100 mg twice daily for 10 days until discharge | Standard care | 28-day all-cause mortality | No | No significant difference in time until discharge alive from hospital or the proportion of patients discharged from hospital alive within 28 days | Not specified | |
Mar - Jul 4, 2020 | 30 countries | 11, 330 (1, 411 in lopinavir group) | Two tablets twice daily for 14 days | The local standard of care | in-hospital mortality | No | Death occurred in 148 of 1, 399 patients receiving lopinavir and in 146 of 1, 372 receiving a control drug (rate ratio, 1.00; 95% CI, 0.79 to 1.25) | Not specified | |
Remdesivir | Feb 21 - Apr 19, 2020 | United States | 1, 062 | Remdesivir 200 mg on day 1, followed by 100 mg daily for up to 9 additional days) | placebo for up to 10 days | Time to clinical recovery | Yes | Patients receiving remdesivir had a significantly shorter median recovery time (10 vs. 15 days) | Not specified |
Mar 15-Apr 18, 2020 | United States, Europe and Asia | 596 | Remdesivir intravenously at 200 mg on day 1 followed by 100 mg/d | Standard care | Clinical status on day 11 on a 7-point ordinal scale | Yes | Patients in the 5-day group had significantly higher odds of a better clinical status distribution | Nausea, hypokalemia, and headache | |
Mar 6 - Mar 26, 2020 | United States and other countries | 397 | Intravenous remdesivir for 5 days | intravenous remdesivir for 10 days | Clinical status on day 14, assessed on a 7-point ordinal scale | No | A clinical improvement of 2 points or more on the ordinal scale occurred in 64% of patients in the 5-day group and in 54% in the 10-day group | Nausea, worsening respiratory failure, elevated alanine aminotransferase level, constipation | |
Feb 6 - Mar 12, 2020 | China | 237 | Remdesivir 200 mg on day 1 followed by 100 mg on days 2-10 in single daily infusions for 10 days | The same volume of placebo infusions for 10 days | Time to a decline of two levels on a six-point ordinal scale of clinical status or discharged from hospital | No | Remdesivir use was not associated with a difference in time to clinical improvement | Constipation, hypoalbuminaemia, hypokalaemia, anaemia, thrombocytopenia, increased total bilirubin | |
Mar 2020 - NA | 30 countries | 11, 330 (2, 750 in remdesivir group) | 200 mg on day 0 and 100 mg intravenously on days 1 through 9 | The local standard of care | in-hospital mortality | No | Death occurred in 301 of 2, 743 patients receiving remdesivir and in 303 of 2, 708 receiving a control agent (rate ratio, 0.95; 95% CI, 0.81 to 1.11) | Not specified | |
Hydroxychloroquine | Mar 25 - Jun 5, 2020 | United Kingdom | 4, 716 | The usual standard of care plus hydroxychloroquine or one of the other available treatments | Usual standard of care | 28-day mortality | No | No significant difference in death within 28 days (27.0% in the hydroxychloroquine group vs. 25.0% in the usual-care group) | Supraventricular tachycardia, atrial flutter |
Mar 29 - May 17, 2020 | Brazil | 667 | Standard care plus hydroxychloroquine at 400 mg twice daily plus azithromycin at 500 mg once daily for 7 days | Standard care; standard care plus hydroxychloroquine at 400 mg twice daily | Clinical status at 15 days as assessed with the use of seven-level ordinal scale | No | The odds of having a higher score at 15 days was not affected by hydroxychloroquine alone (odds ratio: 1.21) or hydroxychloroquine plus azithromycin (odds ratio: 0.99) | Prolongation of the QTc interval, elevated liver enzymes | |
Apr 2 - Jul 17, 2020 | United States | 479 | Hydroxychloroquine (400 mg twice daily for 2 doses, then 200 mg twice daily for 8 doses) | Placebo | Clinical status 14 days after randomization (7-category ordinal scale) | No | Clinical status at 14 days did not significantly differ between the hydroxychloroquine and the placebo groups (median: 6 vs. 6). | QTc interval greater than 500 ms | |
Mar - Jun 19, 2020 | 30 countries | 11, 330 (954 in hydroxychloroquine group) | Four tablets at hour 0, four tablets at hour 6, and starting at hour 12, two tablets twice daily for 10 days | The local standard of care | In-hospital mortality | No | Death occurred in 104 of 947 patients receiving hydroxychloroquine and in 84 of 906 receiving a control agent (rate ratio, 1.19; 95% CI, 0.89 to 1.59) | Not specified | |
Feb 11- Feb 29, 2020 | China | 150 | A loading dose of 1, 200 mg daily for three days followed by a maintenance dose of 800 mg daily (total duration: two or three weeks) | Standard care | Negative conversion by 28 days | No | The probability of negative conversion by 28 days in the standard of care plus hydroxychloroquine group (85.4%%) was similar to that in the standard care group (81.3%) | Diarrhoea being most common; serious adverse events rare | |
Mar 17 - May 6, 2020 | United States and Canada | 821 | Hydroxychloroquine 800 mg once, followed by 600 mg in 6-8 hours, then 600 mg daily for 4 additional days | Placebo | Incidence of lab confirmed Covid-19 or illness compatible with Covid-19 within 14 days | No | The incidence of new illness compatible with Covid-19 did not differ significantly | Nausea, loose stools, abdominal discomfort | |
Mar 17 - Apr 28, 2020 | Spain | 2, 314 | Hydroxychloroquine 800 mg once, followed by 400 mg daily for 6 days | Usual care (no specific therapy) | PCR-confirmed, symptomatic Covid-19 within 14 days | No | Similar incidence of PCR-confirmed, symptomatic Covid-19 in the hydroxychloroquine and usual-care groups (5.7% and 6.2%) | Diarrhea, nausea, abdominal pain, drowsiness, headache | |
Ivermectin | Jul 15 - Dec 21, 2020 | Colombia | 476 | ivermectin 300 μg/kg of body weight per day for 5 days | Placebo for 5 days | Time to resolution of symptoms within 21-day follow-up | No | The median time to resolution of symptoms was 10 days in the ivermectin group compared with 12 days in the placebo group | Headache, dizziness, diarrhea, nausea |
Favipiravir | Feb 4 - Mar 8, 2020 | Iran | 380 | Favipiravir (1.6 gr loading, 1.8 gr daily) | Lopinavir/Ritonavir (800/200 mg daily) | The number of admissions to the intensive care unit | No | The number of deaths, intubations, and ICU admissions were not significantly different | Nausea, anorexia, fatigue, headache |
Interferon beta-1a | Mar 30 - May 30, 2020 | United Kingdom | 101 | 6 MIU interferon beta-1a by inhalation via a mouthpiece daily for 14 days | Placebo | Change in clinical condition on the WHO Ordinal Scale for Clinical Improvement (OSCI) during the dosing period | Yes | Patients receiving SNG001 had greater odds of improvement on the OSCI scale (odds ratio 2.32) on day 15 or 16 and were more likely than those receiving placebo to recover to an OSCI score of 1 (no limitation of activities) during treatment | Headache; few serious adverse events |
Mar - Jul 4, 2020 | 30 countries | 11, 330 (2, 063 in interferon beta-1a group) | Subcutaneous: three doses over 6 days (randomization and days 3 and 6) of 44 μg of interferon beta-1a Intravenous: 10 μg daily for 6 days |
The local standard of care | in-hospital mortality | No | Death occurred in 243 of 2, 050 patients receiving interferon beta-1a and in 216 of 2, 050 receiving its control (rate ratio, 1.16; 95% CI, 0.96 to 1.39) | Not specified | |
Corticosteroids | Mar 19 - Jun 8, 2020 | United Kingdom | 2, 104 | Oral or intravenous dexamethasone (6 mg once daily) for up to 10 days | Usual care | 28-day mortality | Yes | Significantly lower percentage of patients died in the dexamethasone group than in the usual care group (22.9% vs. 25.7%) | Not specified |
Apr 17 - Jun 23, 2020 | Brazil | 299 | Dexamethasone intravenously daily for 5 days, 10 mg dexamethasone daily for 5 days or until ICU discharge, plus standard care | Standard care alone | Ventilator-free days during the first 28 days | Yes | Patients in the dexamethasone group had a mean of 6.6 ventilator-free days vs 4.0 ventilator-free days in the standard care group | Insulin use for glycemia | |
Mar 9 - Jun 17, 2020 | Australia and other countries | 614 | A fixed 7-day course of intravenous hydrocortisone (50 mg or 100 mg every 6 hours) A shock-dependent course (50 mg every 6 hours) | No hydrocortisone | Organ support-free days within 21 days (Bayesian cumulative logistic model) | Yes | The adjusted Bayesian probability of superiority was 93% for the fixed-dose hydrocortisone group | Not specified | |
Mar 7-Jun 1, 202 | France | 149 | Continuous intravenous infusion of hydrocortisone at 200 mg/d at day 1 and continued at 200 mg/d until day 7 and then decreased to 100 mg/d for 4 days and 50 mg/d for 3 days | Placebo (saline) for 14 days | Treatment failure on day 21 | No | No significant difference in the percentage of patients (42.1%) in the hydrocortisone group and in the placebo group (50.7%), who reached the primary endpoint | Not specified | |
Azithromycin | Apr 7 - Nov 27, 2020 | United Kingdom | 16, 442 | Usual standard of care plus azithromycin 500 mg once per day by mouth or intravenously for 10 days or until discharge | Usual standard of care alone for 10 days or until discharge | 28-day all-cause mortality | No | No significant difference in the 28-day all-cause mortality between the azithromycin group and the control group (22% vs. 22%) | Not specified |
May 22 - Nov 30, 2020 | United Kingdom | 2, 265 | Usual care plus azithromycin 500 mg daily for three days | Usual care plus other interventions, or usual care alone | Time to first recovery in 28 days Hospital admission or death related to COVID-19 in 28 days |
No | No significant effect by azithromycin in time to first reported recovery; No significant difference in the percentage of participants being hospitalized or died | Not specified | |
Mar 28 - May 19, 2020 | Brazil | 447 | Azithromycin (500 mg via oral, asogastric, or intravenous administration once daily for 10 days) plus standard of care | Standard of care without macrolides | SARS-CoV-2 infection confirmed by testing before randomisation | No | SARS-CoV-2 infection was not significantly different between the azithromycin and control groups (odds ratio: 1.36) | QTc interval prolongation, gastrointestinal intolerance | |
Baricitinib | May 8- Jul 1, 2020 | United States | 1, 033 | Remdesivir: 200-mg on day 1, 100-mg daily on days 2-10 or until hospital discharge or death Baricitinib: 4-mg daily dose for 14 days or until hospital discharge |
Placebo | Time to recovery within 15 days | Yes | Patients receiving baricitinib experienced a shorter time to recovery and had higher odds of improvement in clinical status at day 15 | Hyperglycemia, anemia, decreased lymphocyte count, acute kidney injury |
Fluvoxamine | Apr 10 - Aug 5, 2020 | United States | 181 | 100 mg of fluvoxamine 3 times daily for 15 days | Placebo 3 times daily for 15 days | Clinical deterioration within 15 days of randomization | Yes | Clinical deterioration occurred in 0 of 80 patients in the fluvoxamine group and in 6 of 72 patients in the placebo group | Pneumonia, nausea, vomiting |
Inhaled budesonide | Jul 16 - Dec 9, 2020 | United Kingdom | 146 | Budesonide dry powder 800 μg twice daily until symptom resolution | Usual care | COVID-19-related urgent care visit | Yes | The primary outcome occurred in 15% participants in the usual care group and 3% in the budesonide group | Sore throat; dizziness |
Ruxolitinib | Feb 9 - Feb 28, 2020 | China | 43 | Ruxolitinib 5 mg twice a day plus standard-of-care treatment | Placebo (100 mg vitamin C) twice daily with standard care | Time to clinical improvement | No | No differences in terms of clinical improvement between ruxolitinib (median: 12 days) and placebo group (median: 15 days) | Anemia, thrombocytopenia, hypercholestrolemia |
Vitamin D | Jun 2 - Aug 27, 2020 | Brazil | 240 | A single oral dose of 200 000 IU of vitamin D3 | A single dose of placebo | Length of stay | No | Median length of stay was not significantly different between the vitamin D3 (7.0 days) and placebo groups (7.0 days) | Not specified |
Drug | Enrollment period | The main countries | No. randomized | Dosing scheme | Control | Primary endpoint | Primary endpoint met | Main findings | Main adverse events |
Tocilizumab | Mar 9 - Nov 19, 2020 | United Kingdom | 755 | Tocilizumab (8 mg per kilogram of body weight) | Standard care alone | Respiratory and cardiovascular organ support-free days to day 21 | Yes | The median number of organ support-free days was 10 in tocilizumab group and 0 in control group (median adjusted cumulative odds ratios: 1.64) | Not specified |
Apr 23, 2020, - Jan 24, 2021 | United Kingdom | 4, 116 | Tocilizumab 400 mg-800 mg given intravenously (the 2nd dose can be given 12-24 h later) plus standard of care | Standard of care alone | 28-day mortality | Yes | 31% of patients allocated tocilizumab and 35% of patients allocated to usual care died within 28 days (rate ratio 0·85; 95% CI 0·76-0·94) | Not specified | |
Apr 20 - Jun 15, 2020 | United States | 243 | Tocilizumab (8 mg per kilogram of body weight administered intravenously, not to exceed 800 mg) | Placebo | Intubation or death, assessed in a time-to-event analysis | No | The hazard ratio for intubation or death in the tocilizumab group compared with the placebo group was 0.83 (95% confidence interval: 0.38 - 1.81) | Neutropenia, elevated liver enzyme | |
Apr 3 - May 28, 2020 | Europe and North America | 452 | A single intravenous infusion of tocilizumab (at a dose of 8 mg per kilogram of body weight) | Placebo intravenous injection | Clinical status at day 28 on an ordinal scale ranging from 1 to 7 | No | The median value for clinical status at day 28 was 1.0 in the tocilizumab group and 2.0 in the placebo group | Infection, bleeding, hypersensitivity | |
May 30 - Aug 31, 2020 | India | 180 | Tocilizumab 6 mg/kg plus standard care | Standard care alone | Progression of COVID-19 up to day 14 | No | Progression of COVID-19 occurred in 9% of patients in the tocilizumab group and 13% in the standard care group (difference −3·71; p=0·42) | Infections | |
Mar 31 - Apr 18, 2020 | France | 131 | Tocilizumb 8 mg/kg, intravenously plus usual care on day 1 and on day 3 if clinically indicated | Usual care alone | Scores higher than 5 on WHO 10-point Clinical Progression Scale on day 4 and survival without ventilation at day 14 | No | In the tocilizumab group, 12 patients vs 19 in the usual care group had scores higher than 5. At day 14, 12% fewer patients needed ventilation or died in the tocilizumab group. | Hepatic cytolysis, anemia, neutropenia | |
Mar 31 - Jun 11, 2020 | Italy | 126 | Intravenous tocilizumab within 8 hours from randomization (8 mg/kg up to 800 mg), followed by a second dose after 12 hours | Supportive care until clinical worsening (could receive tocilizumab) | Entry into intensive care unit with invasive ventilation, death from all causes, or clinical aggravation | No | 28.3% in tocilizumab arm and 27.0% in the standard care group showed clinical worsening within 14 days. Two patients in the tocilzumab group and 1 in the control group died before 30 days; 6 and 5 patients were intubated | Laboratory abnormalities, infection, infestation | |
May 8- Jul 20, 2020 | Brazil | 129 | Tocilizumab (single intravenous infusion of 8 mg/kg) plus standard care | standard care alone | Clinical status measured at 15 days | No | 28% of patients in the tocilizumab group and 20% in the standard care group were receiving mechanical ventilation or died at day 15 (odds ratio, 1.54, P=0.32) | Abnormal liver function, anemia, thrombocytopenia | |
Sarilumab | Jun 20 - Nov 19, 2020 | United Kingdom | 755 | Sarilumab (400 mg) | Standard care alone | Respiratory and cardiovascular organ support-free days up to day 21 | Yes | The median of organ support-free days was 11 in the sarilumab group and 0 in the control group | Not specified |
Mar 28 - Jul 3, 2020 | Argentina and other countries | 431 | intravenous sarilumab 400 mg, sarilumab 200 mg | Placebo | Time to clinical improvement of two or more points (seven point scale) | No | No significant differences in median time to improvement between the placebo (12.0 days) and sarilumab 200 mg (10.0 days) or 400 mg groups (10.0 days) | Increased alanine aminotransferase, invasive bacterial or fungal infection | |
Anakinra | Apr 8- Apr 26, 2020 | France | 116 | Usual care plus anakinra (200 mg twice daily on days 1-3, 100 mg twice on day 4, 100 mg once on day 5) | Usual care alone | Proportion of patients who had died or needed ventilation by day 4; Survival without ventilation at day 14 | No | 36% of patients in the anakinra group had WHO-CPS score > 5 at day 4 versus 38% in the usual care group; 47% patients in the anakinra group and 51% in the usual care group needed ventilation or died |
Acute respiratory distress syndrome, bacterial sepsis, hepatic cytolysis |
Granulocyte colony stimulating factor | Feb 18 - Apr 10, 2020 | China | 199 | 3 doses of recombinant human granulocyte colony-stimulating factor (5 μg/kg, subcutaneously at days 0-2) | Standard care | The time to improvement of at least 1 point on 7-category severity score | No | Time to clinical improvement was similar (median 12 days in the rhG-CSF group vs 13 days in the usual care group; hazard ratio, 1.28; 95% CI, 0.95-1.71) | Neutrophilia, osteodynia, muscle soreness |
Marilimumab | May 28 - Sep 15, 2020 | United States | 40 | Mavrilimumab 6 mg/kg as a single intravenous infusion | Placebo intravenous infusion | The proportion of patients alive and off supplemental oxygen therapy at day 14 | No | Similar percentages of patients (odds Ratio: 1·48, 95% confidence interval: 0·43-5·16) remained alive and were off supplemental oxygen therapy | Bacterial pneumonia |
Vilobelimab | Mar 31 - Apr 24, 2020 | The Netherlands | 30 | Vilobelimab (up to seven doses of 800 mg intravenously) plus best supportive care | Best supportive care | Percentage change in PaO2/FiO2 in supine position between baseline and day 5 | No | No differences between treatment groups (17% change in the vilobelimab group vs 41% in the control group; difference 24% [95% CI: 58 to 9]). | pulmonary embolisms; infections |
Vaccine | Enrollment period | The main countries | Participants | No. randomized | Dosing scheme | Control | Primary endpoint | Vaccine efficacy | Efficacy against variants | Main immunogenicity outcomes | Main safety outcomes |
mRNA-1273 | Apr 16 - May 12, 2020 | United States | Older adults | 40 | Two doses of either 25 μg or 100 μg of vaccine administered 28 days apart | NA | Antibody responses on days 1, 15, 29, 36, 43, and 57 | NA | NA | Anti-S-2P GMT: 25-μg: 323, 945 in 56-70 yr; 1, 128, 391 in ≥71 yr group; 100-μg: 1, 183, 066 and 3, 638, 522 | Predominantly mild or moderate in severity (fatigue, chills, headache, myalgia, pain) |
Jul 27 - Oct 23, 2020 | United States | Individuals 18 years of age or older | 30, 420 | A two-dose regimen containing 0.5 mL injections containing 100 μg of mRNA-1273 | Placebo (saline) | Prevention of Covid-19 with onset > 14 days after 2nd injection in non-infected participants | 94.1% | NA | NA | (Mainly) pain after injection | |
BNT162b1 | May 4 - Jun 22, 2020 | United States | Healthy adults (18-55 yrs; 65-85 yrs) | 195 | Two doses of vaccine (10 μg, 20 μg, 30 μg, and 100 μg), with a 21-day interval; One dose 100 μg of BNT162b1 in one group only | Placebo | Safety ( e.g. , local and systemic reactions and adverse events) | NA | NA | Similar dose-dependent neutralizing GMTs in younger and older adults | Lower incidence and severity of systemic reactions than BNT162b2 |
BNT162b2 | Jul 27, - Nov 14, 2020 | United States and other countries | Adults 16 yrs or older | 43, 548 | BNT162b2 vaccine twice (30 μg per dose), at 21-day interval | Placebo | Efficacy against laboratory-confirmed Covid-19 and safety | 95% | NA | NA | Short-term, mild-to-moderate pain at injection site, fatigue, headache |
Dec 20, 2020, to Feb 1, 2021 | Israel | Non-infected individuals aged 16 yrs or greater | 596, 618 | Having received BNT162b2 vaccination | No vaccination | COVID-19 infection, symptomatic Covid-19, hospitalization, severe illness, death | 7 days after 2nd dose: 92% | NA | NA | NA | |
Dec 8 - Dec 29, 2020 | United Kingdom | Patients with cancer | 151 | Two 30 μg doses of BNT162b2 administered intramuscularly 21 days apart | Single dose of vaccine | Seroconversion to spike protein and vaccine boosting after 21 days on seroconversion | NA | NA | One dose yields poor efficacy. Immunogenicity increased in patients with solid cancer in 2 weeks of boost | No toxicities in 54% patients following the first dose | |
Ad26.COV2.S | Jul 22 - Aug 7, 2020 | Belgium and the United States | Healthy adults aged 18-55 yrs and 65 yrs or greater | 805 | 5×1010 viral particles (low dose); 1×1011 viral particles (high dose) per milliliter |
Placebo | Safety and reactogenicity | NA | NA | Neutralizing antibody detected in ≥90% of participants on day 29 after dose 1 and was 100% by day 57 | NA |
Sep 21, 2020 - Jan 22, 2021 | Argentina, Brazil and other countries | Adults 18-59 yrs and 60 yrs or older | 19, 630 | 5×1010 viral particles as a single intramuscular injection (0.5 mL) | Placebo | Vaccine efficacy against moderate to severe-critical Covid-19 with an onset at least 14 and 28 days | 76.7% at ≥14 days; 85.4% at ≥28 days | 52.0% and 64.0% (20H/501Y. V2 variant) | Higher reactogenicity than with placebo (mild to moderate and transient) | injection-site pain, headache, fatigue, myalgia, nausea | |
Jun 18 - Aug 3, 2020 | Russia | Adults aged 18-60 yrs | 76 | One dose of rAd26-S or one dose of rAd5-S | NA | SARS-CoV-2-specific antibodies on days 0, 14, 21, 28, and 42; number of participants with adverse events | NA | NA | At day 42, RBD IgG titers were 14703 (frozen) and 11 143 (lyophilised) Neutralising antibodies: 49·25 and 45·95, respectively | Common: pain at injection site, hyperthermia, headache, asthenia, muscle and joint pain | |
Jul 29 - Aug 7, 2020 | United States | Participants 18-55 yrs old | 25 | 1 or 2 intramuscular injections with 5 × 1010 viral particles or 1 × 1011 viral particles of Ad26. COV2.S vaccine | Placebo | Cellular immune responses and T cell responses | NA | NA | Binding and neutralizing antibodies emerged by day 8 in 90% and 25% of vaccine recipients | NA | |
Recombinant spike protein nanoparticle vaccine | May 26, - Jun 6, 2020 | Australia | Young and middle-aged adults | 131 | rSARS-CoV-2 vaccine (in 5-μg and 25-μg doses, with or without Matrix-M1 adjuvant) | Placebo | Reactogenicity; serum chemistry, hematology; IgG anti-spike protein response | NA | NA | Two-dose 5-μg regimen induced marked anti-spike IgG and neutralization responses | No serious adverse events; Overall reactogenicity was absent or mild |
Aug 17 - Nov 25, 2020 | South Africa | HIV-positive and -negative adults aged 18-64 yrs | 4, 387 | NVX-CoV2373 (5 μg of recombinant spike protein with 50 μg of Matrix-M1 adjuvant) | Placebo (0.5 mL) | Safety and vaccine efficacy against lab-confirmed symptomatic Covid-19 at 7 days or more after 2nd dose | 60.1% | 51.0% | Vaccine efficacy among HIV-negative participants was 60.1% | Headache, muscle pain, and fatigue most common; no serious adverse events | |
Recombinant spike protein vaccine | Sep 3 - Sep 29, 2020 | Untied States | Adults 18-49 yrs and ≥50 yrs | 441 | One dose (on day 1) or two doses (on days 1 and 22) of placebo or candidate vaccine, containing low dose (effective dose 1·3 μg) or high-dose (2·6 μg) antigen with adjuvant AF03 or AS03 | Unadjuvanted high-dose antigen | Safety up to day 43, and immunogenicity (neutralising antibodies on days 1, 22, and 36) | NA | NA | Neutralizing antibody titers: 13·1 in low-dose + AF03 group, 20·5 in low-dose + AS03 group, 43·2 in high-dose + AF03 group, 75·1 in high-dose + AS03 group | No vaccine-related unsolicited immediate adverse events, serious adverse events |
ChAdOx1 nCoV-19 vaccine | Jun 24 - Nov 9, 2020 | South Africa | Adults 18-65 yrs | 2, 026 | Two doses of vaccine containing 5×1010 viral particles 21 to 35 days apart | Placebo (0.9% saline) | Safety and efficacy against lab-confirmed symptomatic Covid-19 > 14 days after 2nd dose | 21.9% | 21.9% | Strong neutralizing antibodies at 28 days after dose 1 and increased after dose 2 | No serious adverse events |
Apr 23 - May 21, 020 | United Kingdom | Healthy adults aged 18-55 years | 1, 077 | ChAdOx1 nCoV-19 vaccine (5×1010 viral particles) | Menigococcal ACWY vaccine | Cases of symptomatic virologically confirmed COVID-19; Occurrence of serious adverse events |
NA | NA | Anti-spike IgG responses rose by day 28, and were boosted following a second dose | Pain, feverish, chills, muscle ache, headache, malaise; No serious adverse events |
|
May 30 - Aug 8, 2020 | United Kingdom | Adults aged 18-55 yrs, 56-69 yrs, and 70 yrs and older | 560 | intramuscular ChAdOx1 nCoV-19 (2.2×1010 virus particles) | Meningococcal ACWY vaccine | The number of cases of symptomatic, virologically confirmed COVID-19, serious adverse events | NA | NA | Median anti-spike IgG responses 28 days after the boost dose were similar across the three age cohorts | Injection-site pain, feeling feverish, muscle ache, headache (less common in older adults) | |
Apr 23 - Nov 4, 2020 | United Kingdom, Brazil, South Africa | Adults aged 18 yrs and older | 23, 848 | Two doses containing 5×1010 viral particles; A half dose as their first dose; A standard dose as their second dose |
Meningococcal ACWY vaccine | Symptomatic COVID-19 in seronegative participants with PCR-positive swab > 14 days after a second dose | 70.4% | NA | Two standard doses: 62.1%; Low dose followed by standard dose: 90.0% |
Good safety profile with serious adverse events balanced across the study arms | |
Apr 23 - Dec 6, 2020 | United Kingdom, Brazil | individuals aged 18 years and older | 24, 422 | Two standard doses of ChAdOx1 nCoV-19 (5×1010 viral particles) | Control vaccine or placebo | Virologically confirmed symptomatic COVID-19 disease | 66.7% | NA | Antibody levels were maintained with minimal waning by day 90 (geometric mean ratio 0·66 [95%CI 0·59-0·74]) | The most common serious adverse events were infections and infestations | |
May 31 - Dec 30, 2020 | United Kingdom | Healthy people aged 18 years and older | 8, 534 | Standard-dose ChAdOx1 nCoV-19 vaccine (5×1010 viral particles) | Meningococcal conjugate control vaccine | Symptomatic COVID-19 in seronegative participants with positive swab > 14 days after dose 2 | 81.5% for non-B.1.1.7 variant | 70.4% for B.1.1.7 variant | NA | NA | |
BBIBP-CorV | Phase 1: Apr 29 - Jun 28, 2020 Phase 2: May 18 - Jul 30, 2020 |
China | Healthy people Phase 1: 18-80 yrs Phase 2: 18-59 yrs |
640 | A single-dose schedule of 8 μg on day 0; A two-dose schedule of 4 μg on days 0 and 14, 0 and 21, or 0 and 28 |
Placebo | Safety and tolerability | NA | NA | Neutralizing antibody titers on day 28 were greater in the 4 μg days 0 and 14, days 0 and 21, and days 0 and 28 schedules | Mild or moderate; No serious adverse event within 28 days |
Inactivated vaccine | Apr 16 - Apr 25, 020 | China | Adults aged 18-59 years | Phase 1: 144 Phase 2: 743 |
Low dose CoronaVac [3 μg per 0·5 mL of aluminium hydroxide diluent per dose]; High-dose Coronavc [6 μg per 0·5 mL of aluminium hydroxide diluent per dse] |
Placebo | Adverse reactions within 28 days; Seroconversion rates of neutralizing antibodies at day 14 and 28 | NA | NA | Phase 1: Seroconversion rates: 46% in 3μg group, 50% in 6μg group, 0% in placebo group Phase 2: 92%, 98% and 3% |
Phase 1: 29% in the 3μg group, 38% in 6μg group, 8% in the placebo group Phase 2: 33%, 35% and 22% |
July 13 - Jul 30, 2020 | United Kingdom and Brazil | Healthy adults aged 18-55 years | 827 | 3 μg with Algel-IMDG, 6 μg with Algel-IMDG, or 6 μg with Algel | Algel only | Solicited local and systemic reactogenicity events at 2 h and 7 days and throughout the study | NA | NA | Seroconversion rates (%) were 87·9, 91·9, and 82·8 in the 3 μg with Algel-IMDG, 6 μg with Algel-IMDG, and 6 μg with Algel groups | Mild or moderate; more frequent after the first dose | |
May 22 - Jun 1, 2020 | China | healthy adults aged 60 yrs and older | 72 | CoronaVac at 1·5 μg, 3 μg, or 6 μg per dose | Placebo | Adverse reactions within 28; seroconversion rate at 28 days after the second injection | NA | NA | Seroconversion was seen in 90.7% of participants in the 1·5 μg group, 98% in the 3 μg group and 99·0% in the 6 μg group | Mild or moderate in severity; injection site pain was most frequent | |
April 12 - Jul 27, 2020 | China | Healthy adults aged 18-59 yers | Phase 1: 96 Phase 2: 224 |
2.5, 5, and 10 μg/dose inactivated vaccine | Aluminum hydroxide adjuvant | Combined adverse reactions at 7 days Neutralizing antibody response after 14 days | NA | NA | GMTs of neutralizing antibodies in low-, medium-, and high-dose groups at day 14: 316, 206 and 297 in phase 1 | Injection site pain, followed by fever (mild and self-limiting) | |
Non-replicating adenovirus type-5-vectored COVID-19 vaccine | Apr 11 - 16, 2020 | China | Healthy adults aged 18 yrs or older | 603 | 1×1011 viral particles per mL or 5×1010 viral particles per mL | Placebo | GMTs of specific ELISA antibody responses to RBD and neutralising antibody responses at day 28 | NA | NA | The RBD-specific ELISA antibodies peaked at 656·5 and 571·0, with seroconversion rates at 96% and 97% at day 28 | Mostly mild to moderate adverse events; pain being the most common adverse event |
Jun 19 - Sep 23, 2020 | Australia | Younger (18-54 yrs) and older adults (55-75 yrs) | 151 | S-trimer vaccine either 3 μg, 9 μg, or 30 μg | Placebo (0.9% saline) | Safety, tolerability, and immunogenicity of three increasing doses | NA | NA | Fixed doses vaccine induced high titers and seroconversion rates of binding and neutralizing antibodies | Well tolerated overall | |
MF59-adjuvanted subunit vaccine | Jun 23 - Aug 17, 2020 | Australia | Healthy adults (aged ≥18 to ≤55 years) | 314 | Two doses via intramuscular injection 28 days apart of either sclamp vaccine at 5 μg, 15 μg, or 45 μg, or one dose of sclamp vaccine at 45 μg followed by placebo | Placebo | Antigen-specific IgG titer at 28 days; Solicited adverse events in the 7 days Unsolicited events up to 12 months |
NA | NA | Vaccination with SARS-CoV-2 clamp elicited a similar antigen-specific response irrespective of dose | Severe solicited reactions occurred at similar rates in participants receiving vaccines at any dose |
GMT: geometric mean titer; RBD: receptor-binding domain; NAAT: nucleic acid amplification test |
Key unanswered questions related to COVID-19 | Proposed future research directions |
The origin of SARS-CoV-2 and the mode of zoonotic transmissions | To unravel the reservoir of SARS-CoV-2 and understanding the mechanisms of zoonotic infections |
Durability of infectiousness of viral particles in the environment | To investigate the immune escape mechanisms of the emerging variants |
Immunologic mechanisms contributing to the sudden deterioration of clinical status and the clinical recovery | To validate a novel diagnostic method for identifying asymptomatic individuals |
The upper limit of duration of the protective effect of antibodies after natural infection and the receipt of vaccination | To assess the effectiveness of mass vaccination programs in reducing global incidence of COVID-19 |
Factors associated with re-infection | To develop new rapid diagnostic testing for point-of-care use |
Molecular mechanisms underlying prolonged shedding of the live viruses or viral fragments | To identify monoclonal antibody cocktails to improve clinical outcomes |
Association between variants and the efficacy of different types of vaccines | To develop medications that target cellular surface markers in addition to ACE2 |
The mechanisms leading to interstitial lung diseases and the reversibility with immunosuppressants during convalescent phase | To determine the optimal treatment options for non-intubated patients with severe COVID-19 |
The probability and duration of complete recovery after discharge from hospital | To explore new intervention strategies to accelerate the functional and psychological recovery post-discharge from COVID-19 |