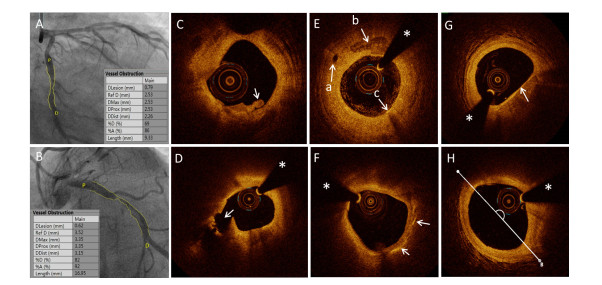
Citation: | Jinxin Liu, Shaohong Fang, Shanjie Wang, Changbin Sun, Rong Sun, Hengxuan Cai, Bo Yu. Effects of intermittent cold-exposure on culprit plaque morphology in ST-segment elevation myocardial infarction patients: a retrospective study based on optical coherence tomography[J]. Frigid Zone Medicine, 2022, 2(2): 82-89. doi: 10.2478/fzm-2022-0011 |
Acute myocardial infarction (AMI) is the primary life-threatening disease worldwide, which is mainly caused by plaque rupture (PR) and plaque erosion (PE)[1]. Different from PE, PR has distinctive vulnerable demographic[2], histology and morphologic characteristics[1, 3]. It posts greater demands for stentimplantation[4] and results in worse outcomes[5]. Thus, more research as well as clinical efforts are needed in combating PR. Optical coherence tomography (OCT) provides a near-pathological diagnosis for identifying and distinguishing PR and PE. OCT can aid to get deeper insight into PR with its capability for accurate morphologic characteristics of plaques[6].
Despite that cold exposure accounts for the high incidence rate of AMI[7-8] and excess winter mortality in wintertime of in frigid zone[9-12], no guideline on how to prevent and reduce cold exposure-induced AMI has been thus far published[13]. While outdoor air temperature or outdoor ambient temperature (OAT) falls down to 4℃ to 0℃, cold exposure is associated with PR[14-15]. The possible association of intermittent cold exposure (ICE) with PR while OAT in wintertime stays below −20℃ remains unclear. The effects of ICE on culprit plaque morphology in patients with ST-segment elevated myocardial infarction (STEMI) in frigid zone remains unknown either. In frigid zone of north-east China, ICE is often the case for people who are frequently alternating their staying in between outdoor cold environment and indoor cozy environment supplied with central heating in the cold seasons. We therefore performed the present study employing OCT technique to explore the association between ICE and culprit plaque morphology in STEMI patients.
A total of 858 patients were enrolled in the present study, who were hospitalized within 24h of symptom onset in the intensive cardiology care unit of the Second Affiliate Hospital of Harbin Medical University between April 2015 and March 2017. The patients all received coronary angiography (CAG) and OCT, of which 10 were excluded because of their fuzzy OCT images. The rest of the patients was then divided into the PR group (N = 637) and the PE group (N = 211), according to the diagnosis based on OCT imaging. Determination of STEMI and the culprit lesion has been described previously[4], and discrimination between PE and PR was done according to the established OCT diagnostic criteria[1]. Thus, PR was defined and categorized as fibrous cap disruption and the presence of thrombus, and PE was defined and categorized as intact intima and the presence of thrombus overlapping culprit lesion. Optimal medical therapy was administered individually based on the current guidelines.
The present study is a retrospective analysis, and the study cohort was selected from the previous EROSION study carried out in our center. It was approved by the Ethics Committee of the Second Affiliated Hospital of Harbin Medical University (approval reference number, KY2017-249), and all patients provided written informed consent prior to their inclusion in the study and the investigation conformed to the principles outlined in the Declaration of Helsinki.
The demographic characteristics of patients including age, gender, hypertension history, diabetes mellitus history, smoking state, and AMI history, lab data including blood cells, admission glucose, hs-CRP, CTnI, creatinine, and lipid profile, and imaging data (CAG and OCT) were collected. In addition, the corresponding OAT data based on 24 solar terms (STs) were also collected by searching the website (http://www.tianqihoubao.com/lishi/haerbin.html).
Quantitative coronary angiography (QCA) was analyzed with the Cardiovascular Angiography Analysis System (CAAS, 5.10, Pie Medical Imaging B.V., Maastricht, the Netherlands). Culprit vessels and lesion sites were identified, and the length of culprit lesion, reference vessel diameter, minimal lesion diameter, and degree of diameter stenosis were measured (Fig. 1A, B).
OCT data were recorded and analyzed using the C7-XR OCT intravascular imaging system (OCT C7 Dragonfly, St. Jude Medical, St. Paul, MN, USA). Quantitative and qualitative analyses of underlying plaques were carried out by two independent operators as described previously[2]. Any discordance was settled by consensus with a third reviewer. Different types of the plaque and thrombus were identified (Fig. 1C, D), along with the identification of microchannels (Fig. 1Ea), spotty calcification (Fig. 1Eb), macrophages (Fig. 1Ec), cholesterol crystals (Fig. 1F), and thin-cap fibroatheroma (TCFA) (Fig. 1G), based on the established diagnostic criteria15. The lesion length, minimal lumen area, minimal thickness of the fibrotic cap, length of the lipid core (LLC), largest arc of the lipid core (LLA) (Fig. 1H), average arc of the lipid core (ALA), and the length of the residual thrombus were measured as described previously[16].
Dichotomous variables are presented as N (%). Continuous variables are presented as mean ± SD or median (P25, P75), and the normality of variables was determined by the Kolmogorov–Smirnov test. For categorical data, chi-squared or Fisher's exact test was applied, and comparisons of continuous data were done by Student's t test for normal variables or the Mann–Whitney test for non-Gaussian variables, as appropriate. Inter-observer reliability and intra-observer reliability were assessed by Kappa statistics. A two-tailed P-value < 0.05 denoted statistical significance.
OAT-related variables involving central heating (Table 1) were grouped according to 24 STs (Fig. 2A): summer (7th–12th STs); intense summer (in-summer; 10–12th STs), winter (19–24th STs), OAT below freezing point 0℃ (ATBFP; 18th STs -6th STs in next year); OAT below −20℃ in severe winter (ATSW, 22–24th STs), and OAT around freezing point (ATAFP) including ATAFP in late spring (ATLS; 4–6th STs), and ATAFP in late autumn (ATLA; 17–19th STs).
Risk weather | ST period | Plaque rupture (N = 637) |
Plaque erosion (N = 211) |
P-value |
Summer | 7–12 | 150 (23.5%) | 43 (20.4%) | 0.341 |
Intense summer | 10–12 | 71 (11.1%) | 19(9%) | 0.381 |
ATBFP | 18-5 | 330 (51.8%) | 119 (56.4%) | 0.247 |
Winter | 10–24 | 157 (24.6%) | 62 (29.4%) | 0.173 |
ATSW | 22–24 | 69 (10.8%) | 36 (17.1%) | 0.017 |
ATAFP | 4–6 and 17–19 | 190(29.8%) | 40(19.0) | 0.002 |
ATLA | 17–19 | 115(18.1%) | 22 (10.4%) | 0.009 |
ATLS | 4–6 | 75 (11.8%) | 18 (8.5%) | 0.191 |
Data are presented as N (%). Abbreviation: ATAFP, air temperature around freezing point; ATBFP, air temperature below freezing point; ATLA, air temperature around freezing point in late autumn; ATLS, air temperature arround freezing point in late spring; ATSW, air temperature in severe winter; ST, solar term. |
Univariate and multivariate logistic regression analyses were used to screen the OAT groups which were significantly associated with culprit plaque type. A P < 0.05 was considered statistically significant. ROC curve analysis was applied to evaluate the predictive ability of the OAT variables. Subsequently, the variables associated significantly with culprit plaque type were included in a differential diagnostic model to evaluate their diagnosis value. Furthermore, the correlation between the OAT predictive factors and plaque morphology was analyzed with spearman correlation. The analyses were produced by SPSS 20.0 Software.
Central heating began supplying when OAT fell to about 0℃, usually from 15 October (in ATLA) to 15 April (in ATLS) the following year to keep indoor temperature about 25℃. During either ATLA or ATLS, PR incidence increased gradually until reaching a summit. It declined in ATLA after central heating units began operating but increased in ATLS after central heating ceased supplying till OAT climbing up above 0℃. There was a bottom PR incidence and a peak of PE incidence during ATSW while central heating was supplied constantly (Fig. 2).
Differences in OAT-related variables between PR and PE are summarized in Table 1. For example, ATAFP (P = 0.002) and ATSW (P = 0.017) were significantly different between PR and PE. Yet, stratification analysis pointed to only ATLA with significant inter-group difference (P = 0.009), and no significant inter-group difference was found in summer and intense summer, nor in ATBFP and winter.
Logistic regression analysis revealed significant association of ATAFP and ATSW with culprit plaque type. PR was positively and significantly associated with ATAFP, whereas PE was positively and significantly associated with ATSW. No significant PR-PE differences were associated with other OAT-related variables. The association of PR and PE with their respective OAT-related variables remained even after adjustment for the risk factors associated with plaque, including age, gender, DM history, HTN history, and current smoking. Intriguingly, with serum LDL-C, creatinine and ARPG being taken into consideration for adjustment, the significant association between PR and ATAFP remained while that between PE and ATSW disappeared. Adjustment to culprit vessel and multivessel lesion did not change the association of PR with ATAFP. Stratification analysis confirmed the significant difference between PR and ATLA, but not between PR and ATLS, no matter whether being adjusted or not (Fig. 3A).
The association between PE and ATSW within subgroups was then analysed (Fig. 3B). Logistic regression analysis showed positive and significant association of PE with ATSW only in patients > 50 years old, female, or current smokers. No significant association was identified among the STEMI patients whether or not with DM or HTN history. After adjusting for the risk factors associated with plaque model, the association was weakened but reserved in patients who were female or age over 50 years but was broken in current smokers.
Subsequently, we quantified the diagnostic accuracy of ATLA for PR and ATSW for PE using the receiver operating characteristic curve (ROC). The results indicated that the area under curve (AUC) was not sufficiently large to determine its diagnostic power. However, when ATLA or ATSW was combined with various risk factors (age, gender, smoking, DM, hypertension, creatine, admission glucose and LDL-C) to establish combined predictive models for culprit plaque morphology, the area under curve (AUC) was significantly increased. Moreover, when culprit vessel and multivessel lesion were added to the combinations, the AUCs for both ATLA and ATSW exceeded 70% (AUC=0.706 for ATLA and 0.704 for ATSW) (Fig. 4).
Furthermore, the correlations between temperature-related variables and imaging data of the STEMI patients were analyzed by Spearman's correlation analysis. The results uncovered a negative correlation of summer and ATLS with the size of lipid arc, minimal FCT and TCFA. ATBFP including ATSW presented positive and weak correlation with the size of lipid arc, minimal FCT and TCFA. Subgroup analysis showed that ATLS was negatively and weakly correlated with the size of lipid arc, minimal FCT and TCFA, while ATEW had positive correlations with these factors (Fig. 5).
The present study is the first to explore the association between ICE and culprit plaque morphology in STEMI patients based on OCT imaging analyses. The major findings include: (1) PR incidence showed a peak value during ATLA and declined gradually in winter, which was affected by ICE. Persistent cold exposure in ATLA was an independent predictor for PR in STEMI patients. (2)PE incidence peaked in the coldest days during ATSW with constant central heating supply. ICE in ATSW was an independent predictor for PE in STEMI patients > 50 years old, female, or non-current smoker. (3)The effect of ICE on offender plaque morphology was attributable to interruption of cold exposure and thus reduced the lipid size and TCFA in culprit vessel in STEMI patients.
Different from the published data[14], our results showed that PR incidence did not peak in the coldest days in ATEW; instead, it peaked during ATLA and ATLS. PR incidence declined gradually in ATLA following the supply of central heating and climbed up in ATLA after ceasing of central heating supply. The association between PR and cold OAT in ATSW dissipated with constant supply of central heating. Our data support that persistent cold exposure was the major cause of PR, and ICE contributed to preventing vulnerable patients from PR. ICE was established with the introduction of central heating unit that broke persistent cold exposure and might reduce PR by preventing vulnerable patients from psychological distress[17], arterial pressure rise[18], blood coagulation[19], and respiratory infections[20] imposed by cold exposure. Cold exposure is a risk factor for the development of atherosclerotic plaque[21], the main cause of STEMI. This implies that reducing PR due to ICE could well minimize excess winter mortality associated with cold exposure[22] and insufficient protection[23-24].
Different from PR, PE incidence increased and reached a peak value during ATSW when OAT dropped below −20℃, even with reduced time or interruption of cold-exposure with central heating. The decline of PR might lead to a relative increase of PE, but it could not explain why PE presented the highest annual incidence in ATSW. A published study suggested that PR might transform to PE when damage persists and plaque repair surpasses damage[25]. It appears that the culprit plaque model in STEMI patients might undergo transformation from PR to PE with ICE. We therefore postulated that the peak of PE incidence in severe winter might partly be ascribed to an imbalance of damage-repair of the vulnerable plaque. Staying outdoor with cold-exposure to −20℃ or below during ATSW in frigid zone can damage the vulnerable plaques; in contrast, staying indoor with temperature kept constantly at around 25℃ by central heating helps repair the vulnerable plaques. With ICE, vulnerable plaques undergo repeated damages and repairs. Such a process stimulates the transformation of PR to PE progressively and imperceptibly. Yet, this hypothesis and the pathophysiological mechanisms behand the postulated process require rigorous scrutinization and examination in future studies.
The present study represents the first to unravel that cold weather was positively correlated with lipid size and TCFA in culprit vessel reported by OCT images. Lipid deposition is a characteristic lesion of PR and TCFA[26-27], which is correlated well with the retention and accumulation of oxidation of LDL, a causal factor for the development of vulnerable plaques in the culprit vessel of STEMI patients[28-29]. Given that cold exposure is associated with the increase of plasma cholesterol concentration as a key contributor to thrombosis[18], ICE might change the culprit plaque model via decreasing lipid size and TCFA in culprit vessel. The culprit plaque differentiation of AMI caused by ICE may partly benefited from its role in improving glucose homeostasis[30]. Our findings lay the groundwork for further studies on the pathogenesis of PR and PE.
Our findings might also help stratifying STEMI patients, selecting the treatment strategy, and improving the prognosis of them in winter. The results also address that the duration of central heating supply with OAT below 0℃ is not long enough to prevent or minimize the peak of PR. It should be prolonged to cover the whole ATAFP, and better protective measures should be applied to the vulnerable patients during the period. The vulnerable patients, who are over 50 years old, female, or current smokers, are the population in need of more close attention in order to be better protected from adverse events. Lipid-rich plaque, TCFA and PR[5] are positively associated with higher adverse clinical outcomes[31]. Thus, enhanced lipid-regulating therapy should be seriously implemented for residents living in frigid zones, particularly the vulnerable patients with possible cold exposure.
We fully recognized the limitations of the present study. First, it was a single center observational study based on retrospective design. Aiming to explore the relationship between OAT-related variables and culprit plaque morphology of STEMI, two-year qualified population was recruited consecutively based on the qualitative and quantitative analysis of CAG and OCT images. Second, 10 patients were excluded because of the poor quality of OCT image, making the sample size therefore not all patients with STEMI caused by PR and PE were succeeding to be included.
In conclusion, the present study first demonstrated that ICE was associated with PR incidence reduction and the transformation from PR to PE in STEMI patients in winter. Persistent cold exposure in ATLA was an independent predictor for PR, and ICE in ATSW for PE in STEMI patients in residential areas equipped with central heating in cold winter. ICE might impact the culprit model of STEMI via reducing lipid accumulation and developing TCFA. Prolonging central heating properly and strengthen protective measure might reduce the occurrence of STEMI caused by either PR or PE in severe cold residential area.
Bo Yu is an Editorial Board Member of the journal. The article was subject to the journal's standard procedures, with peer review handled independently of him and his research groups.
This work was supported by the National Key R&D Program of China (Grant No. 2016YFC1301100; awarded to B.Y.), the National Natural Fund Program of China (Grant NO.81827806; awarded to B.Y.), and the Province Natural Fund Key Program of Shandong Province, China (Grant No. ZR2020KH028; awarded to J-X.L.).
We sincerely thank all the personnel working in the interventional catheter room of the Second Affiliated Hospital of Harbin Medical University.
The present study is a retrospective one involving the patients from the EROSION study of our center previously. The study protocol was approved by the Ethics Committee of the Second Affiliated Hospital of Harbin Medical University (KY2017-249), and all patients provided written informed consent prior to the inclusion in the study and the investigation conformed to the principles outlined in the Declaration of Helsinki.
Bo Yu contributed to conceptualization, funding acquisition, project administration, supervision, resources, and writing - review & editing; Jinxin Liu contributed to conceptualization, funding acquisition, investigation, methodology; formal analysis, validation; visualization; roles/writing - original draft, and writing - review & editing; Hengxuan Cai, Rong sun, Changbin Sun, and Shaohong Fang contributed to investigation, data curation, and validation; Shanjie Wang contributed to investigation, software, formal analysis, and methodology.
[1] |
Jia H, Abtahian F, Aguirre A D, et al. In vivo diagnosis of plaque erosion and calcified nodule in patients with acute coronary syndrome by intravascular optical coherence tomography. J Am Coll Cardiol, 2013; 62(19): 1748-1758. doi: 10.1016/j.jacc.2013.05.071
|
[2] |
Dai J, Xing L, Jia H, et al. In vivo predictors of plaque erosion in patients with st-segment elevation myocardial infarction: A clinical, angiographical, and intravascular optical coherence tomography study. Eur Heart J, 2018; 39(22): 2077-2085. doi: 10.1093/eurheartj/ehy101
|
[3] |
Virmani R, Kolodgie F D, Burke A P, et al. Lessons from sudden coronary death: A comprehensive morphological classification scheme for atherosclerotic lesions. Arterioscler, Thromb, and Vasc biol, 2000; 20(5): 1262-1275. doi: 10.1161/01.ATV.20.5.1262
|
[4] |
Jia H, Dai J, Hou J, et al. Effective anti-thrombotic therapy without stenting: Intravascular optical coherence tomography-based management in plaque erosion (the erosion study). Eur Heart J, 2017; 38(11): 792-800.
|
[5] |
Niccoli G, Montone R A, Di Vito L, et al. Plaque rupture and intact fibrous cap assessed by optical coherence tomography portend different outcomes in patients with acute coronary syndrome. Eur Heart J, 2015; 36(22): 1377-1384. doi: 10.1093/eurheartj/ehv029
|
[6] |
Yabushita H, Bouma B E, Houser S L, et al. Characterization of human atherosclerosis by optical coherence tomography. Circulation, 2002; 106(13): 1640-1645. doi: 10.1161/01.CIR.0000029927.92825.F6
|
[7] |
Spencer F A, Goldberg R J, Becker R C, et al. Seasonal distribution of acute myocardial infarction in the second national registry of myocardial infarction. J Am Coll Cardiol, 1998; 31(6): 1226-1233. doi: 10.1016/S0735-1097(98)00098-9
|
[8] |
Sun Z, Chen C, Xu D, et al. Effects of ambient temperature on myocardial infarction: A systematic review and meta-analysis. Environ Pollut, 2018; 241: 1106-1114. doi: 10.1016/j.envpol.2018.06.045
|
[9] |
Sheth T, Nair C, Muller J, et al. Increased winter mortality from acute myocardial infarction and stroke: The effect of age. J Am Coll Cardiol, 1999; 33(7): 1916-1919 doi: 10.1016/S0735-1097(99)00137-0
|
[10] |
Rumana N, Kita Y, Turin T C, et al. Seasonal pattern of incidence and case fatality of acute myocardial infarction in a japanese population (from the takashima ami registry, 1988 to 2003). Am J Cardiol, 2008; 102(10): 1307-1311. doi: 10.1016/j.amjcard.2008.07.005
|
[11] |
Vasconcelos J, Freire E, Almendra R, et al. The impact of winter cold weather on acute myocardial infarctions in portugal. Environ Pollut, 2013; 183: 14-18. doi: 10.1016/j.envpol.2013.01.037
|
[12] |
Ogbebor O, Odugbemi B, Maheswaran R, et al. Seasonal variation in mortality secondary to acute myocardial infarction in England and Wales: A secondary data analysis. BMJ Open, 2018; 8(7): e019242. doi: 10.1136/bmjopen-2017-019242
|
[13] |
Davie G S, Baker M G, Hales S, et al. Trends and determinants of excess winter mortality in new zealand: 1980 to 2000. BMC Public Health, 2007; 7: 263. doi: 10.1186/1471-2458-7-263
|
[14] |
Shibuya J, Kobayashi N, Asai K, et al. Comparison of coronary culprit lesion morphology determined by optical coherence tomography and relation to outcomes in patients diagnosed with acute coronary syndrome during winter -vs- other seasons. Am J Cardiol, 2019; 124(1): 31-38. doi: 10.1016/j.amjcard.2019.03.045
|
[15] |
Prati F, Regar E, Mintz G S, et al. Expert review document on methodology, terminology, and clinical applications of optical coherence tomography: Physical principles, methodology of image acquisition, and clinical application for assessment of coronary arteries and atherosclerosis. Eur Heart J, 2010; 31(4): 401-415. doi: 10.1093/eurheartj/ehp433
|
[16] |
Liu X, Sun C, Gu X, et al. Intraplaque neovascularization attenuated statin benefit on atherosclerotic plaque in cad patients: A follow-up study with combined imaging modalities. Atherosclerosis, 2019; 287: 134-139. doi: 10.1016/j.atherosclerosis.2019.06.912
|
[17] |
Sher L. Effects of seasonal mood changes on seasonal variations in coronary heart disease: Role of immune system, infection, and inflammation. Med Hypotheses, 2001; 56(1): 104-106. doi: 10.1054/mehy.2000.1122
|
[18] |
Keatinge W R, Coleshaw S R, Cotter F, et al. Increases in platelet and red cell counts, blood viscosity, and arterial pressure during mild surface cooling: Factors in mortality from coronary and cerebral thrombosis in winter. Br Med J, 1984; 289(6456): 1405-1408. doi: 10.1136/bmj.289.6456.1405
|
[19] |
Chen X, Shang W, Huang X, et al. The effect of winter temperature on patients with ischemic stroke. Med Sci Monit, 2019; 25: 3839-3845. doi: 10.12659/MSM.916472
|
[20] |
Mattila K J, Valtonen V V, Nieminen M S, et al. Role of infection as a risk factor for atherosclerosis, myocardial infarction, and stroke. Clin Infect Dis, 1998; 26(3): 719-734. doi: 10.1086/514570
|
[21] |
Sun Z, Cade R, Morales C. Role of central angiotensin ii receptors in cold-induced hypertension. Am J Cardiol. 2002; 15(1 Pt 1): 85-92.
|
[22] |
Barnett A G, Sans S, Salomaa V, et al. The effect of temperature on systolic blood pressure. Blood Press Monit, 2007; 12(3): 195-203. doi: 10.1097/MBP.0b013e3280b083f4
|
[23] |
Cold exposure and winter mortality from ischaemic heart disease, cerebrovascular disease, respiratory disease, and all causes in warm and cold regions of europe. The eurowinter group. Lancet, 1997; 349(9062): 1341-1346. doi: 10.1016/S0140-6736(96)12338-2
|
[24] |
Donaldson G C, Ermakov S P, Komarov Y M, et al. Cold related mortalities and protection against cold in yakutsk, eastern siberia: Observation and interview study. BMJ. 1998; 317(7164): 978-982. doi: 10.1136/bmj.317.7164.978
|
[25] |
Liu J, Wang S, Hou J, et al. Proteomics profiling reveals insulinlike growth factor 1, collagen type vi alpha-2 chain, and fermitin family homolog 3 as potential biomarkers of plaque erosion in st-segment elevated myocardial infarction. Cir J, 2020; 84(6): 985-993. doi: 10.1253/circj.CJ-19-1206
|
[26] |
Virmani R, Burke A P, Kolodgie F D, et al. Vulnerable plaque: The pathology of unstable coronary lesions. J Inter Card, 2002; 15(6): 439- 446. doi: 10.1111/j.1540-8183.2002.tb01087.x
|
[27] |
Virmani R, Burke A P, Farb A, et al. Pathology of the vulnerable plaque. J Am Coll Cardiol, 2006; 47(Suppl 8): C13-18.
|
[28] |
Tulenko T N, Sumner A E. The physiology of lipoproteins. Journal of Nuclear Cardiology, 2002; 9(6): 638-649. doi: 10.1067/mnc.2002.128959
|
[29] |
Williams K J, Tabas I. The response-to-retention hypothesis of early atherogenesis. Arterioscler Thromb Vasc Biol, 1995; 15(5): 551-561. doi: 10.1161/01.ATV.15.5.551
|
[30] |
McKie G L, Shamshoum H, Hunt K L, et al. Intermittent cold exposure improves glucose homeostasis despite exacerbating dietinduced obesity in mice housed at thermoneutrality. J Physiol, 2021; 600(4): 829-845.
|
[31] |
Amano H, Noike R, Yabe T, et al. Frailty and coronary plaque characteristics on optical coherence tomography. Heart Vessels, 2020; 35(6): 750-761. doi: 10.1007/s00380-019-01547-2
|
[1] | Zhiwei Zhang, Yongjin Long, Ming Li, Chunpeng Lyu, Xianglan Chen, Qiaoyu Wang, Kunying Yang, Jiahui Li, Wei Zhang, Dianjun Sun. SPSB4 as a risk factor for papillary thyroid cancer: Enhancing cell vitality under excess iodine exposure[J]. Frigid Zone Medicine, 2025, 5(1): 22-41. doi: 10.1515/fzm-2025-0003 |
[2] | Xu Wang, Hongbo Hu, Ying Zhang. Identification of hub genes and pathways in mouse with cold exposure[J]. Frigid Zone Medicine, 2024, 4(4): 233-241. doi: 10.1515/fzm-2024-0023 |
[3] | Tongzhu Jin, Zhen Ye, Ruonan Fang, Yue Li, Wei Su, Qianqian Wang, Tianyu Li, Hongli Shan, Yanjie Lu, Haihai Liang. Notum protects against myocardial infarction-induced heart dysfunction by alleviating cardiac fibrosis[J]. Frigid Zone Medicine, 2024, 4(1): 41-50. doi: 10.2478/fzm-2024-0005 |
[4] | Lina Xuan, Huishan Luo, Shu Wang, Guangze Wang, Xingmei Yang, Jun Chen, Jianjun Guo, Xiaomeng Duan, Xiufang Li, Hua Yang, Shengjie Wang, Hailong Zhang, Qingqing Zhang, Shulei Liu, Yongtao She, Kai Kang, Lihua Sun. Circulating CCRR serves as potential novel biomarker for predicting acute myocardial infarction[J]. Frigid Zone Medicine, 2024, 4(3): 137-151. doi: 10.1515/fzm-2024-0015 |
[5] | Miao Yan, Shanjie Wang, Shaohong Fang, Mingyan E, Bo Yu. Impacts of cold exposure on energy metabolism[J]. Frigid Zone Medicine, 2024, 4(2): 65-71. doi: 10.1515/fzm-2024-0007 |
[6] | Yanan Ni, Dan Liu, Xiaona Zhang, Hong Qiao. Association of point in range with β-cell function and insulin sensitivity of type 2 diabetes mellitus in cold areas[J]. Frigid Zone Medicine, 2023, 3(4): 242-252. doi: 10.2478/fzm-2023-0031 |
[7] | Xiangyu Bian, Xi Li, Tong Xu, Li Zhang, Yongqiang Zhang, Shuai Wu, Renren Yang, Weiyun Dong, Changjiang Guo, Danfeng Yang, Weina Gao. Cold exposure alters proteomic profiles of the hypothalamus and pituitary in female rats[J]. Frigid Zone Medicine, 2023, 3(2): 114-125. doi: 10.2478/fzm-2023-0015 |
[8] | Guanqun Li, Le Li. The intricate relationship between cold exposure and gut microbiota[J]. Frigid Zone Medicine, 2023, 3(3): 131-133. doi: 10.2478/fzm-2023-0018 |
[9] | Tong Zhao, Xin Liu. Acute cold exposure triggers thermogenic memory in brown adipose tissue[J]. Frigid Zone Medicine, 2023, 3(4): 199-201. doi: 10.2478/fzm-2023-0026 |
[10] | Pingping Tang, Henghui Xu, Yong Zhang. Unveiling metabolic flux changes during acute cold exposure[J]. Frigid Zone Medicine, 2023, 3(4): 193-195. doi: 10.2478/fzm-2023-0024 |
[11] | Manyu Gong, Xuewen Yang, Yaqi Wang, Yanying Wang, Dongping Liu, Haodong Li, Yunmeng Qu, Xiyang Zhang, Yanwei Zhang, Han Sun, Lei Jiao, Ying Zhang. Growth differentiation factor 11 promotes macrophage polarization towards M2 to attenuate myocardial infarction via inhibiting Notch1 signaling pathway[J]. Frigid Zone Medicine, 2023, 3(1): 53-64. doi: 10.2478/fzm-2023-0008 |
[12] | Gaosong Wu, Yuhao Zhang, Ningning Zheng, Saisai Tian, Jingyu Liao, Wanqi Le, Houkai Li, Weidong Zhang. Cold exposure promotes coronavirus infection by altering the gut microbiota and lipid metabolism to reduce host immunity[J]. Frigid Zone Medicine, 2023, 3(4): 216-231. doi: 10.2478/fzm-2023-0029 |
[13] | Yongqiang Zhang, Li Zhang, Shuai Wu, Guanyu Zhang, Xiaodie Wei, Xi Li, Danfeng Yang. Silicon dioxide nanoparticles inhibit the effects of cold exposure on metabolism and inflammatory responses in brown adipocytes[J]. Frigid Zone Medicine, 2023, 3(2): 97-104. doi: 10.2478/fzm-2023-0013 |
[14] | Tingting Li, Yue Yuan. Moderate cold exposure contributes to the extension of lifespan by alleviating the aggregation of disease-related proteins[J]. Frigid Zone Medicine, 2023, 3(3): 129-130. doi: 10.2478/fzm-2023-0017 |
[15] | Xin Xing, Shiqiang Wang. Mammalian hibernation: a unique model for medical research[J]. Frigid Zone Medicine, 2021, 1(2): 65-68. doi: 10.2478/fzm-2021-0008 |
[16] | Jia Chen, Jinghua Luo, Hao Liu, Xue Du, Shan Zhang, Zizhen Wang, Liu He, Zhichun Feng. Ibuprofen treatment for patent ductus arteriosus in preterm infants: a retrospective cohort study in a leading Chinese center[J]. Frigid Zone Medicine, 2021, 1(2): 103-110. doi: 10.2478/fzm-2021-0013 |
[17] | Yan Feng, Ying Li, Xinling Yang, Limin Han, Luning Wang, Shan Gao, Ruixue Yin, Xue Wang, Jiayang Li, Meiming Liu, Baiyan Li. Direct evidence of VEGF-mediated neuroregulation and afferent explanation of blood pressure dysregulation during angiogenic therapy[J]. Frigid Zone Medicine, 2021, 1(2): 119-126. doi: 10.2478/fzm-2021-0015 |
[18] | Hui Yu, Yun Zhou, Yu Duan, Yunlong Gao, Ning Fang, Jiawei Zhang, Yue Li. Tranilast treats cold-related hypertension by reducing the expression of NLRP3 inflammasome[J]. Frigid Zone Medicine, 2021, 1(2): 95-101. doi: 10.2478/fzm-2021-0012 |
[19] | De-an Guo, Wenlong Wei, Changliang Yao, Jianqing Zhang, Qirui Bi. Chinese herbal medicines with beneficial effects[J]. Frigid Zone Medicine, 2021, 1(2): 79-83. doi: 10.2478/fzm-2021-0010 |
[20] | Yanyan Liu, Yahan Yu, Xinyao Wang, Guanqun Liu, Xinda Yin, Yunlong Bai, Zhimin Du. Overexpression of microRNA-135b-5p attenuates acute myocardial infarction injury through its antioxidant and anti-apptotic properties[J]. Frigid Zone Medicine, 2021, 1(2): 85-94. doi: 10.2478/fzm-2021-0011 |
Risk weather | ST period | Plaque rupture (N = 637) |
Plaque erosion (N = 211) |
P-value |
Summer | 7–12 | 150 (23.5%) | 43 (20.4%) | 0.341 |
Intense summer | 10–12 | 71 (11.1%) | 19(9%) | 0.381 |
ATBFP | 18-5 | 330 (51.8%) | 119 (56.4%) | 0.247 |
Winter | 10–24 | 157 (24.6%) | 62 (29.4%) | 0.173 |
ATSW | 22–24 | 69 (10.8%) | 36 (17.1%) | 0.017 |
ATAFP | 4–6 and 17–19 | 190(29.8%) | 40(19.0) | 0.002 |
ATLA | 17–19 | 115(18.1%) | 22 (10.4%) | 0.009 |
ATLS | 4–6 | 75 (11.8%) | 18 (8.5%) | 0.191 |
Data are presented as N (%). Abbreviation: ATAFP, air temperature around freezing point; ATBFP, air temperature below freezing point; ATLA, air temperature around freezing point in late autumn; ATLS, air temperature arround freezing point in late spring; ATSW, air temperature in severe winter; ST, solar term. |